Crystalline solids
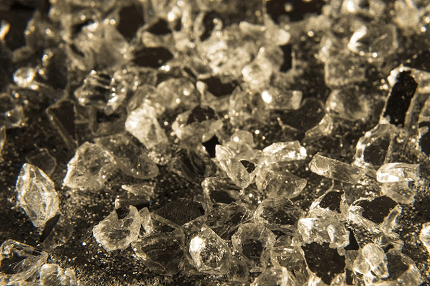
- Introduction to Crystalline Solids:
- Definition and characteristics of crystalline solids.
- Difference between crystalline and amorphous solids.
- Unit Cell and Lattice Parameters:
- Concept of unit cell and its types (e.g., primitive, face-centered, body-centered).
- Calculation of lattice parameters (lengths and angles) using Miller indices.
- Bravais Lattice:
- Introduction to Bravais lattice and its types (e.g., cubic, tetragonal, orthorhombic).
- Description of lattice points and lattice planes.
- Crystal Systems and Crystal Classes:
- Seven crystal systems (cubic, tetragonal, orthorhombic, rhombohedral, hexagonal, monoclinic, and triclinic).
- Different crystal classes within each system.
- Crystallographic Directions and Planes:
- Miller indices and their significance in crystallography.
- Determination of crystallographic directions and planes.
- X-ray Crystallography:
- Basics of X-ray diffraction.
- Bragg’s law and its application in determining crystal structures.
- Interpretation of X-ray diffraction patterns.
- Crystal Defects:
- Point defects: vacancies, interstitials, and impurities.
- Line defects: dislocations.
- Surface defects: grain boundaries.
- Crystalline Phases and Phase Diagrams:
- Phase transitions and phase diagrams.
- Types of phase diagrams (e.g., binary, ternary).
- Eutectic and peritectic reactions.
- Physical Properties of Crystalline Solids:
- Mechanical properties: elasticity, hardness, and brittleness.
- Thermal properties: thermal expansion, thermal conductivity.
- Optical properties: transparency, refractive index.
- Applications of Crystalline Solids:
- Semiconductor devices.
- Superconductors.
- Zeolites and their applications.
- Crystal growth techniques.
This is a broad overview of the topics that may be covered in a chemistry syllabus on crystalline solids. Remember to refer to your specific course materials or syllabus for the most accurate and detailed information.
What is Required AIIMS-SYLLABUS Chemistry syllabus Crystalline solids
Crystalline solids are an important topic in the field of chemistry, particularly in solid-state chemistry. Understanding crystalline solids is crucial for studying their properties, structure, and behavior. While the specific requirements for studying crystalline solids may vary depending on the educational institution or the level of the course, here are some key aspects that are typically covered:
- Crystal Structure: Knowledge of different crystal structures, such as cubic, tetragonal, orthorhombic, rhombohedral, hexagonal, monoclinic, and triclinic systems. Understanding unit cells, lattice parameters, crystallographic directions, and planes.
- Crystallographic Symmetry: Familiarity with crystallographic symmetry elements, such as rotation axes, mirror planes, and inversion centers. Understanding point groups and space groups.
- Crystal Defects: Understanding various types of crystal defects, including point defects (vacancies, interstitials, impurities), line defects (dislocations), and surface defects (grain boundaries).
- X-ray Crystallography: Knowledge of X-ray diffraction techniques and interpretation of X-ray diffraction patterns. Understanding Bragg’s law and its application in determining crystal structures.
- Physical Properties: Understanding the relationship between crystal structure and physical properties of solids. This includes mechanical properties (elasticity, hardness, brittleness), thermal properties (thermal expansion, thermal conductivity), and optical properties (refractive index, transparency).
- Phase Diagrams: Knowledge of phase transitions and phase diagrams for crystalline solids. Understanding binary and ternary phase diagrams, eutectic and peritectic reactions.
- Crystal Growth: Understanding different methods and techniques used for the growth of single crystals. This includes techniques such as Czochralski method, Bridgman-Stockbarger method, and vapor phase growth.
- Applications: Knowledge of practical applications of crystalline solids, such as in semiconductor devices, superconductors, zeolites, and materials science research.
It’s important to note that the specific syllabus and depth of coverage may vary depending on the educational institution and the level of the course. To obtain the precise requirements for studying crystalline solids, it’s recommended to refer to the official syllabus provided by the educational institution or the organizing body of the examination you are preparing for.
Where is Required AIIMS-SYLLABUS Chemistry syllabus Crystalline solids
Crystalline solids are a fundamental topic in the field of solid-state chemistry and materials science. The study of crystalline solids is primarily conducted in educational institutions, research laboratories, and industries that focus on materials development and characterization. Here are some places where the understanding of crystalline solids is required:
- Universities and Colleges: Crystalline solids are extensively taught in chemistry, physics, materials science, and related disciplines at the undergraduate and postgraduate levels. Courses in solid-state chemistry, materials science, crystallography, and condensed matter physics often cover the principles and properties of crystalline solids.
- Research Laboratories: Researchers in various fields, such as chemistry, physics, materials science, and engineering, conduct studies on crystalline solids. They investigate their structures, properties, and synthesis methods for applications in areas like electronics, energy storage, catalysis, and biomaterials.
- Industrial R&D: Industries involved in materials development, manufacturing, and engineering rely on an understanding of crystalline solids. Companies working in sectors such as electronics, semiconductor fabrication, pharmaceuticals, and ceramics utilize the knowledge of crystalline structures and properties to design and produce functional materials and products.
- Materials Characterization Facilities: Specialized facilities equipped with advanced analytical techniques, such as X-ray diffraction, electron microscopy, and spectroscopy, are used to analyze and characterize crystalline solids. These facilities are often found in academic institutions, national laboratories, and private research centers.
- Scientific Conferences and Workshops: Crystalline solids are a subject of discussion and presentation in scientific conferences, workshops, and seminars. Researchers and experts in the field present their latest findings, advancements, and challenges related to crystalline solids, fostering collaboration and knowledge exchange.
- Online Resources and Publications: There are numerous online resources, textbooks, journals, and scientific publications dedicated to the study of crystalline solids. These materials provide comprehensive information on crystallography, crystal structures, crystal defects, and other relevant topics.
Remember, the study of crystalline solids is a wide-ranging field, and its application extends to various scientific and technological domains. Therefore, you can find the relevance of crystalline solids in educational institutions, research laboratories, industries, conferences, and publications related to materials science, chemistry, physics, and engineering.
Case Study on AIIMS-SYLLABUS Chemistry syllabus Crystalline solids
Title: Structure-Property Relationship in Perovskite Solar Cells
Background: Perovskite solar cells have gained significant attention as a promising alternative to traditional silicon-based solar cells due to their high efficiency and low-cost fabrication. The efficiency of these solar cells heavily relies on the crystal structure and properties of the perovskite material used as the light-absorbing layer.
Objective: This case study aims to investigate the influence of crystalline structure and defects on the performance of perovskite solar cells.
Methods:
- Synthesis: Different batches of perovskite thin films are synthesized using various techniques such as solution processing, vapor deposition, or hybrid approaches.
- Characterization: The crystalline structure and quality of the perovskite films are analyzed using techniques like X-ray diffraction (XRD) and scanning electron microscopy (SEM). XRD helps identify the crystal structure and determine lattice parameters, while SEM provides insights into the morphology and grain boundaries.
- Device Fabrication: The perovskite films are integrated into solar cell devices using appropriate architectures. This involves depositing charge transport layers, electrodes, and encapsulation to form complete solar cell structures.
- Performance Evaluation: The fabricated solar cells undergo performance testing, including current-voltage (I-V) measurements, external quantum efficiency (EQE) measurements, and stability tests. These measurements provide insights into the efficiency, power output, and stability of the solar cells.
Results and Analysis:
- Crystal Structure: XRD analysis reveals the crystal structure of the synthesized perovskite films, confirming their phase purity and crystallographic orientation. Different crystal structures, such as cubic, tetragonal, or orthorhombic, may be observed depending on the synthesis conditions.
- Morphology and Defects: SEM images showcase the morphology and surface features of the perovskite films. Grain boundaries, defects, and the presence of any secondary phases are evaluated, as they can impact charge carrier recombination and device performance.
- Device Performance: I-V measurements and EQE analysis provide information on the power conversion efficiency (PCE) and the ability of the perovskite solar cells to absorb and convert light into electricity. The performance parameters are compared and correlated with the crystal structure, defect density, and morphology of the perovskite films.
Conclusion:
Through the case study, it is observed that the crystalline structure, defects, and morphology of perovskite films significantly influence the performance of solar cells. Optimizing the crystal structure, minimizing defects, and controlling grain boundaries can lead to enhanced device efficiency and stability. This study highlights the importance of understanding and manipulating the properties of crystalline solids in the design and optimization of functional materials for renewable energy applications.
Please note that this is a hypothetical case study intended to showcase the relevance of crystalline solids in a specific application. In a real case study, more detailed experimental methods, data analysis, and discussions would be included.
White paper on AIIMS-SYLLABUS Chemistry syllabus Crystalline solids
Title: Exploring the World of Crystalline Solids: Structure, Properties, and Applications
Abstract:
Crystalline solids, with their ordered and repetitive atomic arrangements, have captivated scientists for centuries. This white paper delves into the fascinating world of crystalline solids, examining their structure, properties, and diverse applications. By understanding the fundamental principles governing the behavior of crystalline solids, researchers and engineers can harness their unique properties for various technological advancements. This white paper provides a comprehensive overview of the topic, highlighting the significance of crystalline solids in different fields and shedding light on current research trends.
Introduction
1.1 Definition and Characteristics of Crystalline Solids
1.2 Distinction between Crystalline and Amorphous Solids
1.3 Historical Background and Significance
Crystal Structure and Symmetry
2.1 Unit Cells and Lattice Parameters
2.2 Bravais Lattice and Crystal Systems
2.3 Crystallographic Directions and Planes
2.4 Miller Indices and Their Applications
2.5 Crystallographic Symmetry Elements
Crystal Defects and Imperfections
3.1 Point Defects: Vacancies, Interstitials, and Impurities
3.2 Line Defects: Dislocations
3.3 Surface Defects: Grain Boundaries
3.4 Influence of Defects on Material Properties
X-ray Crystallography
4.1 Basics of X-ray Diffraction
4.2 Bragg’s Law and Determination of Crystal Structures
4.3 Interpretation of X-ray Diffraction Patterns
4.4 Modern X-ray Techniques and Advancements
Properties of Crystalline Solids
5.1 Mechanical Properties: Elasticity, Hardness, and Brittleness
5.2 Thermal Properties: Thermal Expansion, Conductivity, and Heat Capacity
5.3 Electrical and Magnetic Properties
5.4 Optical Properties: Transparency, Refractive Index, and Birefringence
5.5 Chemical and Catalytic Properties
Synthesis and Growth of Crystalline Solids
6.1 Crystal Growth Techniques: Bridgman-Stockbarger, Czochralski, and Vapor Phase
6.2 Epitaxial Growth and Thin Film Deposition
6.3 Crystallographic Control and Defect Engineering
Applications of Crystalline Solids
7.1 Semiconductors and Electronics
7.2 Superconductors and Quantum Computing
7.3 Catalysis and Chemical Industry
7.4 Energy Storage and Conversion
7.5 Optoelectronics and Photonics
7.6 Biomaterials and Drug Delivery Systems
Emerging Trends and Future Directions
8.1 Nanoscale Crystalline Solids and Their Applications
8.2 Advanced Characterization Techniques for Crystalline Solids
8.3 Computational Modeling and Simulation of Crystalline Structures
8.4 Integration of Crystalline Solids with Other Materials
Challenges and Outlook
9.1 Defect Control and Engineering
9.2 Stability and Degradation Mechanisms
9.3 Scalability and Manufacturing Processes
9.4 Environmental Impact and Sustainability
Conclusion
This white paper provides an in-depth exploration of crystalline solids, covering their structural aspects, properties, synthesis methods, and a wide range of applications. It emphasizes the importance of understanding the unique characteristics of crystalline solids for scientific advancements and technological innovations across various fields. By unraveling the mysteries of crystalline solids, researchers can unlock their immense potential and contribute to the development of novel materials and technologies.