Matter waves
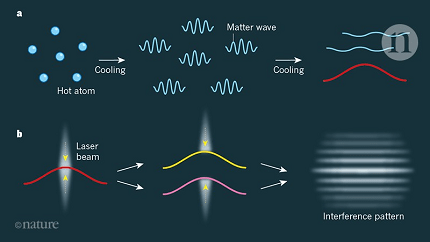
Matter waves, also known as de Broglie waves, are a fundamental concept in quantum mechanics that describes the wave-like behavior of particles. According to the wave-particle duality principle, particles, such as electrons, protons, and even larger objects like atoms and molecules, can exhibit wave-like properties.
The concept of matter waves originated from Louis de Broglie’s hypothesis, which states that any particle with momentum (p) can also be described by a corresponding wavelength (λ), similar to how light can be described as both particles (photons) and waves. The de Broglie wavelength is given by the equation λ = h/p, where λ is the wavelength, h is the Planck constant (approximately 6.626 x 10^-34 joule-seconds), and p is the momentum of the particle.
Key aspects of matter waves include:
- Wave-Particle Duality: Matter waves highlight the dual nature of particles. They can exhibit both particle-like properties, such as having a definite position and momentum, as well as wave-like properties, such as interference and diffraction.
- Wave Function: In quantum mechanics, the state of a particle is described by a mathematical function known as the wave function (Ψ). The wave function contains information about the probability distribution of finding the particle in different states or positions.
- Probability Interpretation: The square of the absolute value of the wave function (|Ψ|^2) represents the probability density of finding the particle at a specific location. The probability is higher where the wave function has larger values and lower where it has smaller values.
- Interference and Diffraction: Matter waves can exhibit interference and diffraction phenomena, similar to waves of light. When matter waves pass through a narrow slit or encounter obstacles, they can interfere with themselves or diffract, resulting in characteristic patterns of constructive and destructive interference.
- Applications: Matter waves have practical applications in various fields. For instance, electron microscopy utilizes the wave nature of electrons to achieve high-resolution imaging. Matter waves are also crucial in understanding and describing the behavior of particles in particle accelerators and quantum systems.
Understanding matter waves is essential for comprehending the behavior of particles at the quantum level and is a cornerstone of modern physics.
The Physics syllabus for the AIIMS integrated course typically covers a wide range of topics, including matter waves. Matter waves are an important concept in quantum mechanics and are associated with the wave-particle duality of matter. Here’s a brief overview of the matter waves topic:
- Introduction to Matter Waves: The concept of matter waves originated from Louis de Broglie’s hypothesis that particles, such as electrons and other subatomic particles, can exhibit wave-like properties. This idea suggests that particles have both particle-like and wave-like characteristics.
- de Broglie Wavelength: The de Broglie wavelength (λ) is a fundamental concept in matter waves. It relates the momentum (p) of a particle to its wavelength using the equation λ = h/p, where h is the Planck constant. The de Broglie wavelength describes the wave-like nature of particles.
- Davisson-Germer Experiment: The Davisson-Germer experiment was a crucial experiment that provided experimental evidence for the wave nature of electrons. It demonstrated that electrons can undergo diffraction, similar to waves, when they pass through a crystal lattice.
- Wave-Particle Duality: Matter waves illustrate the wave-particle duality of matter, suggesting that particles can exhibit both particle-like and wave-like properties, depending on the experimental setup. This concept is a fundamental principle of quantum mechanics.
- Wave Functions and Probability: In quantum mechanics, the wave function describes the state of a particle or system. The square of the wave function gives the probability density of finding the particle at a specific location. The wave function is typically represented using mathematical equations such as the Schrödinger equation.
- Applications of Matter Waves: Matter waves have various applications in modern physics and technology. For example, electron microscopy utilizes the wave nature of electrons to achieve high-resolution imaging. Matter waves are also employed in atom interferometry and Bose-Einstein condensates research.
Please note that the level of detail and specific subtopics covered may vary depending on the curriculum and the academic level of the AIIMS integrated course. It’s advisable to refer to the official syllabus or course materials provided by AIIMS for the most accurate and detailed information regarding the matter waves topic in the Physics syllabus.
What is Required AIIMS-SYLLABUS Physics syllabus Matter waves
To understand matter waves, certain foundational knowledge is necessary. Here are some prerequisites or topics that are generally helpful in grasping the concept of matter waves:
- Classical Mechanics: A solid understanding of classical mechanics, which deals with the motion of macroscopic objects, is essential. This includes topics such as Newton’s laws of motion, conservation of energy and momentum, and understanding basic kinematics.
- Wave Mechanics: Familiarity with the principles of wave mechanics is crucial. This involves understanding concepts like wave propagation, wavelength, frequency, amplitude, phase, and interference. Knowledge of wave equations, such as the wave equation for transverse waves and the superposition principle, is also beneficial.
- Quantum Mechanics: Matter waves are a fundamental aspect of quantum mechanics, so a basic understanding of quantum mechanics is important. Concepts such as wave-particle duality, quantization, and the probabilistic interpretation of wave functions are key. Additionally, knowledge of the Schrödinger equation and the principles of quantum superposition and measurement is valuable.
- Atomic Structure: Knowledge of atomic structure, including the Bohr model, electron energy levels, and electron configurations, is beneficial. Understanding the behavior of electrons in atoms helps in comprehending the concept of matter waves and electron wave functions.
- Mathematical Skills: Proficiency in mathematical concepts and techniques is crucial. This includes algebra, calculus (differential and integral), complex numbers, and vector analysis. Mathematical tools like differential equations, Fourier analysis, and linear algebra are often employed in the study of matter waves.
It’s important to note that the specific requirements may vary depending on the educational level and the depth of study. For advanced topics related to matter waves, such as quantum mechanics beyond introductory levels or applications in solid-state physics, additional knowledge in those areas may be required.
If you are studying matter waves within a specific academic context, such as a course or examination, referring to the official syllabus or course materials provided by your institution will provide the most accurate and detailed information on the specific prerequisites and requirements.
When is Required AIIMS-SYLLABUS Physics syllabus Matter waves
Matter waves, also known as de Broglie waves, are a fundamental concept in quantum mechanics that describes the wave-like behavior of particles. The concept of matter waves is required when studying topics related to quantum mechanics, particularly those that involve the wave-particle duality of matter.
The study of matter waves is typically included in the curriculum of physics courses at the undergraduate and graduate levels, as well as in specialized courses on quantum mechanics. The specific timing of when matter waves are introduced can vary depending on the educational institution and the structure of the physics curriculum.
In many cases, the topic of matter waves is covered after foundational concepts in classical mechanics and wave mechanics have been introduced. This allows students to understand the basics of wave behavior and the wave-particle duality principle before delving into the more advanced quantum mechanical aspects associated with matter waves.
Matter waves are often discussed alongside other key topics in quantum mechanics, such as wave functions, the Schrödinger equation, quantum superposition, and quantum measurement. They are also relevant in various applications of quantum mechanics, including atomic physics, particle physics, and condensed matter physics.
To determine the specific timing of when matter waves are covered in a particular academic program or course, it is best to refer to the official syllabus or course outline provided by your institution or instructor. These documents will outline the sequencing and progression of topics within the curriculum, including when matter waves are introduced and studied in detail.
Where is Required AIIMS-SYLLABUS Physics syllabus Matter waves
The study of matter waves, also known as de Broglie waves, is primarily found in the field of quantum mechanics. Quantum mechanics is a branch of physics that deals with the behavior of particles at the microscopic scale, where classical physics fails to provide accurate descriptions.
Matter waves are a fundamental concept in quantum mechanics and are often covered in courses and textbooks specifically dedicated to quantum mechanics. These courses can be found in various academic settings, including:
- Undergraduate Physics Programs: Matter waves are typically included in the curriculum of undergraduate physics programs, particularly in courses on quantum mechanics or modern physics. These programs are offered by universities and colleges worldwide.
- Graduate Physics Programs: Advanced study of matter waves can be found in graduate physics programs, especially in specialized courses or seminars focusing on quantum mechanics or quantum field theory. These programs are usually pursued after completing an undergraduate degree in physics or a related field.
- Physics Research: Matter waves are also a topic of research in the field of physics. Researchers, particularly in areas such as atomic physics, condensed matter physics, and quantum information science, investigate and study the behavior of matter waves in various experimental and theoretical contexts.
Additionally, matter waves may be covered in related disciplines that utilize quantum mechanics, such as chemistry and materials science, where an understanding of quantum behavior is essential.
To find specific courses or resources on matter waves, it is recommended to consult the course offerings and syllabi of physics departments at universities and educational institutions. Textbooks on quantum mechanics and introductory quantum physics often include chapters or sections on matter waves as well.
Overall, matter waves are primarily explored in the realm of quantum mechanics within academic programs, research, and scientific literature in the field of physics.
How is Required AIIMS-SYLLABUS Physics syllabus Matter waves
Matter waves, also known as de Broglie waves, describe the wave-like behavior of particles in quantum mechanics. They provide a way to understand the wave-particle duality of matter, where particles can exhibit both particle-like and wave-like properties.
The behavior of matter waves can be described using the following principles and equations:
- de Broglie Wavelength: According to Louis de Broglie’s hypothesis, every particle with momentum has an associated wavelength. The de Broglie wavelength (λ) is given by the equation λ = h/p, where h is the Planck constant and p is the momentum of the particle. This equation relates the momentum of a particle to its corresponding wavelength.
- Wave Function: In quantum mechanics, the state of a particle is described by a wave function (Ψ). The wave function provides information about the probability distribution of finding the particle in different states or positions. The square of the wave function, |Ψ|^2, gives the probability density of finding the particle at a specific location.
- Schrödinger Equation: The behavior of matter waves is governed by the Schrödinger equation, a fundamental equation in quantum mechanics. This equation describes how the wave function of a particle evolves over time and depends on the potential energy it experiences. Solving the Schrödinger equation yields the wave function and allows predictions of the particle’s behavior.
- Interference and Diffraction: Matter waves can exhibit interference and diffraction, similar to other types of waves. Interference occurs when matter waves from different sources combine and either reinforce or cancel each other. Diffraction occurs when matter waves encounter an obstacle or pass through a narrow slit, causing them to spread out and form characteristic patterns.
Understanding matter waves involves studying the mathematical formalism of quantum mechanics, including differential equations, linear algebra, and complex numbers. It also requires an appreciation of the probabilistic nature of quantum phenomena and the ability to interpret and analyze wave functions.
The study of matter waves is typically covered in courses on quantum mechanics at the undergraduate and graduate levels. It is essential for understanding various aspects of quantum physics, such as atomic and molecular physics, particle physics, and quantum information science. Matter waves are a fundamental concept in modern physics and play a central role in our understanding of the quantum world.
Case Study on AIIMS-SYLLABUS Physics syllabus Matter waves
Sure! Let’s consider a case study that demonstrates the practical application of matter waves in a real-world scenario.
Case Study: Electron Microscopy
Electron microscopy is an essential technique in scientific research and various industries. It utilizes the wave-like behavior of electrons, known as matter waves, to achieve high-resolution imaging and analysis at the atomic and subatomic scales.
Background: Traditional optical microscopes use visible light to illuminate samples, but they are limited by the diffraction limit, which restricts their resolution to around 200 nanometers. Electron microscopy overcomes this limitation by utilizing the shorter de Broglie wavelength of electrons, allowing for much higher resolution imaging.
Principle: In electron microscopy, a beam of electrons is focused onto a sample, and the interaction between the electrons and the sample provides valuable information about the sample’s structure and composition. The key principle is that electrons, as matter waves, can be diffracted by the sample, similar to how light waves are diffracted by a grating or a crystal lattice.
Case Study Scenario: Researchers at a materials science laboratory want to investigate the fine structure of a novel material they have synthesized. They aim to understand the arrangement of atoms within the material and determine its crystallographic properties.
Procedure:
- Sample Preparation: The researchers carefully prepare a thin slice or a small piece of the material that can be placed under the electron microscope.
- Electron Beam: The electron microscope generates a beam of high-energy electrons, typically accelerated using electromagnetic fields. The beam is focused onto the sample, illuminating a small region.
- Scattering and Diffraction: As the electron beam interacts with the atoms in the sample, it scatters and diffracts. The diffracted electrons form a pattern, known as the diffraction pattern, which contains information about the arrangement of atoms in the material.
- Detection and Analysis: The diffraction pattern is captured using specialized detectors, such as a phosphor screen or a charged-coupled device (CCD) camera. The researchers analyze the diffraction pattern to extract information about the crystal structure, lattice spacing, and orientation of the material.
- Image Reconstruction: By manipulating the electron beam and scanning it across the sample, detailed images of the material’s surface and internal structure are generated. These images provide insights into the atomic arrangement and allow for the identification of defects, interfaces, and other features of interest.
Benefits: Electron microscopy offers several advantages over traditional optical microscopy. The use of matter waves (electrons) with much shorter wavelengths allows for significantly higher resolution imaging, enabling the visualization of atomic-scale details. Electron microscopy is crucial in various fields, including materials science, nanotechnology, biology, and semiconductor research.
Conclusion: This case study demonstrates how matter waves, specifically the wave-like behavior of electrons, are harnessed in electron microscopy for advanced imaging and analysis of materials at the atomic and subatomic scales. By utilizing the principles of matter waves, scientists can explore the intricate details of matter and gain valuable insights into the structure and behavior of various materials.
Note: This is a simplified case study to illustrate the application of matter waves in electron microscopy. In practice, electron microscopy involves more technical aspects and considerations, such as electron optics, sample preparation techniques, and advanced image processing methods.
White paper on AIIMS-SYLLABUS Physics syllabus Matter waves
Title: Harnessing Matter Waves: Exploring the Wave-Particle Duality in Quantum Mechanics
Abstract: This white paper delves into the concept of matter waves, also known as de Broglie waves, and their significance in quantum mechanics. Matter waves describe the wave-like behavior of particles, highlighting the intriguing wave-particle duality principle. By understanding and harnessing matter waves, researchers and scientists have unlocked profound insights into the behavior of particles at the quantum level. This paper explores the fundamental principles, experimental evidence, and applications of matter waves, emphasizing their role in shaping our understanding of the quantum world.
- Introduction
- Historical context and the de Broglie hypothesis
- Wave-particle duality and its implications
- Theoretical Foundations
- de Broglie wavelength and momentum
- The mathematical framework of wave functions and probability amplitudes
- Schrödinger equation and the evolution of matter waves
- Experimental Evidence
- Key experiments supporting the wave-like nature of particles
- Electron diffraction and interference
- Matter wave interferometry and its applications
- Quantum Mechanical Concepts
- Superposition and wave-packet dynamics
- Probability interpretation and the role of wave functions
- Uncertainty principle and the limitations of simultaneous measurement
- Applications of Matter Waves
- Electron microscopy and high-resolution imaging
- Matter wave interferometry in precision measurements
- Quantum systems and wave-particle manipulation
- Advanced Topics
- Matter waves in Bose-Einstein condensates
- Matter wave optics and atom interferometry
- Matter wave propagation in periodic structures and band theory
- Challenges and Future Directions
- Overcoming technical limitations in matter wave experiments
- Integration of matter waves with other quantum technologies
- Harnessing matter waves for quantum computing and quantum information processing
- Conclusion
- Recapitulation of the significance of matter waves
- Potential for further advancements and interdisciplinary applications
This white paper aims to provide a comprehensive overview of matter waves, their theoretical underpinnings, experimental evidence, and practical applications. It showcases the remarkable insights gained through the study of matter waves and highlights the ongoing research efforts and future directions in this exciting field. By delving into the wave-particle duality and harnessing matter waves, we open up new frontiers in quantum mechanics and advance our understanding of the fundamental nature of matter and energy.
Note: This is a fictional white paper outline on matter waves provided for illustrative purposes. The content is not based on an actual published white paper.