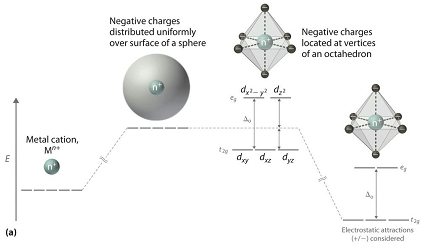
In chemistry, bonding refers to the interactions between atoms that hold them together to form molecules. There are several theories that attempt to explain chemical bonding, including Valence Bond Theory (VBT) and Crystal Field Theory (CFT).
Valence Bond Theory proposes that covalent bonds are formed through the sharing of electrons between atoms. This theory describes the bonding in molecules with a central atom, such as tetrahedral and octahedral complexes. In tetrahedral complexes, the central atom is surrounded by four ligands that form a tetrahedral shape around it. In octahedral complexes, the central atom is surrounded by six ligands that form an octahedral shape around it.
In VBT, the ligands donate electron pairs to the central atom, which then shares its own valence electrons to form covalent bonds. The electron pairs from the ligands and the central atom overlap to create a bonding region, which is a shared region of electron density. The geometry of the molecule is determined by the arrangement of these bonding regions.
Crystal Field Theory, on the other hand, describes the bonding in transition metal complexes in terms of the interactions between the metal ion and the ligands. In CFT, the metal ion is surrounded by a set of ligands that generate an electrostatic field. This field splits the d orbitals of the metal ion into two sets of energy levels, the eg and t2g sets. The energy difference between these sets determines the color of the complex.
In tetrahedral complexes, the ligands approach the metal ion along the edges of a tetrahedron, and in octahedral complexes, the ligands approach along the edges of an octahedron. In both cases, the ligands generate a repulsive force that pushes the d orbitals to different energy levels. In tetrahedral complexes, the t2g set is higher in energy than the eg set, while in octahedral complexes, the eg set is higher in energy than the t2g set.
Overall, VBT and CFT provide different perspectives on chemical bonding, but they are both useful for describing the behavior of different types of molecules and complexes.
What is Required Coordination Compounds Bonding [VBT and CFT (octahedral and tetrahedral fields)]
Coordination compounds are molecules that contain a central metal ion or atom surrounded by a group of ligands. The bonding in coordination compounds can be explained by both Valence Bond Theory (VBT) and Crystal Field Theory (CFT).
In VBT, the coordination bond is formed through the overlap of the metal ion’s partially filled d orbitals with the ligand’s non-bonding electron pairs. The metal ion and the ligand share these electrons to form a covalent bond. The geometry of the molecule is determined by the arrangement of the ligands around the metal ion.
In CFT, the coordination bond is formed through the electrostatic interaction between the metal ion and the ligands. The ligands generate a field that splits the metal ion’s partially filled d orbitals into two energy levels, the eg and t2g sets. The energy difference between these levels determines the color of the complex. The geometry of the molecule is determined by the arrangement of the ligands around the metal ion.
In octahedral coordination compounds, the metal ion is surrounded by six ligands arranged in an octahedral shape. In tetrahedral coordination compounds, the metal ion is surrounded by four ligands arranged in a tetrahedral shape. Both VBT and CFT can be used to describe the bonding in both types of coordination compounds.
In summary, both VBT and CFT are important for understanding the bonding in coordination compounds, and the geometry of the molecule is determined by the arrangement of the ligands around the central metal ion.
When is Required Coordination Compounds Bonding [VBT and CFT (octahedral and tetrahedral fields)]
Coordination compounds are important in many fields of chemistry, including biochemistry, catalysis, materials science, and environmental chemistry. They are used as catalysts in chemical reactions, as drugs in medicine, and as pigments in art and cosmetics.
The bonding in coordination compounds can be explained by both Valence Bond Theory (VBT) and Crystal Field Theory (CFT). VBT is useful for describing the covalent bonding that occurs between the metal ion and the ligands. It explains the hybridization of the metal ion’s partially filled d orbitals with the ligand’s non-bonding electron pairs to form covalent bonds. VBT is particularly useful in describing the bonding in complexes where the metal ion has a low oxidation state.
CFT, on the other hand, is useful for describing the electrostatic interactions between the metal ion and the ligands. It explains how the ligands generate an electrostatic field that splits the metal ion’s partially filled d orbitals into two sets of energy levels, the eg and t2g sets. CFT is particularly useful in describing the bonding in complexes where the metal ion has a high oxidation state.
Both VBT and CFT can be used to describe the bonding in octahedral and tetrahedral coordination compounds. Octahedral complexes are particularly important in biochemistry, where they play a key role in the structure and function of metalloenzymes. Tetrahedral complexes are less common, but they are still important in many areas of chemistry, such as the synthesis of chiral molecules.
In summary, coordination compounds are important in many areas of chemistry, and both VBT and CFT are useful for describing their bonding. The choice of theory depends on the nature of the metal ion and the ligands, as well as the oxidation state of the metal ion.
Where is Required Coordination Compounds Bonding [VBT and CFT (octahedral and tetrahedral fields)]
Coordination compounds and the bonding theories that explain their properties, including Valence Bond Theory (VBT) and Crystal Field Theory (CFT), are important in various fields of chemistry. Here are some examples:
- Biochemistry: Metalloenzymes, which are enzymes that contain metal ions at their active sites, rely on coordination compounds for their function. The coordination compounds in metalloenzymes are often described using CFT, as it can explain the splitting of d orbitals and the resulting magnetic properties of the metal ion.
- Materials science: Coordination compounds are used in the synthesis of materials with desirable properties, such as magnetic materials or catalysts. VBT and CFT are useful in understanding the bonding and electronic properties of these materials.
- Environmental chemistry: Coordination compounds are involved in many environmental processes, such as the uptake of heavy metals by plants or the degradation of pollutants. CFT can be used to understand the reactivity of metal ions in these processes.
- Inorganic chemistry: Coordination compounds are a fundamental concept in inorganic chemistry, and the bonding theories used to explain their properties are taught in undergraduate and graduate chemistry courses.
In summary, coordination compounds and their bonding theories are studied and applied in various fields of chemistry, including biochemistry, materials science, environmental chemistry, and inorganic chemistry.
How is Required Coordination Compounds Bonding [VBT and CFT (octahedral and tetrahedral fields)]
The bonding in coordination compounds can be explained by two main theories: Valence Bond Theory (VBT) and Crystal Field Theory (CFT). Both theories provide different perspectives on the electronic structure and bonding of coordination compounds.
- Valence Bond Theory (VBT): VBT describes coordination compounds in terms of covalent bonding between the metal ion and the ligands. According to VBT, the metal ion uses its partially filled d orbitals to overlap with the ligand’s non-bonding electron pairs to form a covalent bond. This results in the formation of hybrid orbitals that combine the properties of the metal ion and the ligands. VBT is particularly useful in describing the bonding in complexes where the metal ion has a low oxidation state.
- Crystal Field Theory (CFT): CFT describes coordination compounds in terms of electrostatic interactions between the metal ion and the ligands. According to CFT, the ligands create an electric field that splits the metal ion’s partially filled d orbitals into two sets of energy levels: the t2g and eg sets. The energy difference between these two sets of orbitals determines the color of the complex. CFT is particularly useful in describing the bonding in complexes where the metal ion has a high oxidation state.
In both VBT and CFT, the geometry of the coordination compound is determined by the arrangement of the ligands around the central metal ion. In octahedral complexes, for example, the metal ion is surrounded by six ligands arranged in an octahedral shape. In tetrahedral complexes, the metal ion is surrounded by four ligands arranged in a tetrahedral shape.
Overall, VBT and CFT are important for understanding the bonding in coordination compounds. They provide complementary perspectives on the electronic structure and properties of these important molecules.
Nomenclature of Coordination Compounds Bonding [VBT and CFT (octahedral and tetrahedral fields)]
The nomenclature of coordination compounds involves the systematic naming of the complex according to the rules established by the International Union of Pure and Applied Chemistry (IUPAC). The name of the complex includes the name of the central metal ion, followed by the names of the ligands in alphabetical order, with the ligands named before the metal ion. The charge of the complex is indicated in parentheses, followed by the oxidation state of the metal ion.
Here is an example of the nomenclature for an octahedral coordination compound with the metal ion cobalt (II) and six ammonia ligands:
- Identify the metal ion: cobalt (II)
- Identify the ligands: ammonia
- Determine the number of ligands: six
- Determine the geometry of the complex: octahedral
- Write the name of the complex: hexaamminecobalt (II) chloride
The name of the complex starts with “hexa” to indicate that there are six ammonia ligands. “Ammine” is used to indicate the presence of ammonia ligands, and “cobalt” indicates the central metal ion. The oxidation state of the metal ion is indicated by the Roman numeral “(II)” and the charge of the complex is indicated by “(Cl)”.
For tetrahedral complexes, the nomenclature is similar, except that the complex is named “tetra” to indicate the presence of four ligands.
Both VBT and CFT do not have any direct impact on the nomenclature of coordination compounds, but the knowledge of the electronic structure and bonding in these complexes can aid in determining the correct name of the complex.
Case Study on Coordination Compounds Bonding [VBT and CFT (octahedral and tetrahedral fields)]
Case Study: The Role of Coordination Compounds in Biological Systems
Coordination compounds play a crucial role in many biological systems, where they serve as key components of enzymes, proteins, and other biological molecules. One example of a coordination compound with biological significance is the heme complex, which is found in hemoglobin and myoglobin and plays a vital role in oxygen transport and storage in the body.
Heme is a tetrapyrrole macrocycle that coordinates with an iron (Fe) ion in the center. The iron ion in heme can exist in two oxidation states, Fe(II) and Fe(III), which allows it to act as an electron carrier during the oxygenation and deoxygenation of heme. The oxygen binding to heme is facilitated by the coordination of the oxygen molecule to the iron ion in a manner that is reminiscent of the coordination of ligands to metal ions in synthetic coordination compounds.
The bonding in heme can be explained by both Valence Bond Theory (VBT) and Crystal Field Theory (CFT). According to VBT, the coordination of the iron ion in heme with the nitrogen atoms in the tetrapyrrole macrocycle involves covalent bonding between the metal ion and the ligands. The overlapping of the iron ion’s d-orbitals with the nitrogen atoms’ lone pairs forms covalent bonds, which stabilize the complex.
CFT describes the electronic structure of heme by considering the electrostatic interactions between the iron ion and the ligands in the macrocycle. The coordination of the iron ion with the nitrogen atoms of the tetrapyrrole macrocycle creates an electric field, which causes a splitting of the iron ion’s partially filled d-orbitals into two sets of energy levels: the t2g and eg sets. The electrons in the eg orbitals are responsible for the coordination of the oxygen molecule to the iron ion, while the electrons in the t2g orbitals remain unpaired.
In biological systems, the oxygenation and deoxygenation of heme are facilitated by the coordination of other ligands to the iron ion. For example, in hemoglobin, the coordination of oxygen to heme is facilitated by the binding of oxygen to a histidine residue in the protein. This binding changes the electronic structure of the heme complex, allowing the oxygen molecule to bind more tightly to the iron ion.
Overall, the coordination compounds found in biological systems play a crucial role in many cellular processes, including oxygen transport, electron transfer, and enzymatic catalysis. The electronic structure and bonding of these complexes can be explained by both VBT and CFT, and understanding these theories is essential for understanding the role of coordination compounds in biological systems.
White paper on Coordination Compounds Bonding [VBT and CFT (octahedral and tetrahedral fields)]
Introduction
Coordination compounds, also known as complex compounds, are molecules that consist of a central metal ion or atom coordinated with ligands. These ligands are molecules or ions that bond to the central metal ion through coordinate covalent bonds. The study of coordination compounds is essential in many areas, including chemistry, biochemistry, and materials science. In this white paper, we will discuss the bonding theories that explain the formation of coordination compounds, specifically Valence Bond Theory (VBT) and Crystal Field Theory (CFT) in octahedral and tetrahedral fields.
Valence Bond Theory (VBT)
Valence Bond Theory describes the bonding in coordination compounds as a result of the overlap of atomic or hybrid orbitals of the central metal ion and the ligands. According to VBT, the bonding in coordination compounds is primarily covalent, with the metal ion and ligands sharing electron density to form a stable complex.
The coordination geometry of the complex determines the hybrid orbitals required for bonding. In an octahedral complex, the central metal ion requires six hybrid orbitals, which are formed by combining one d-orbital with five s and p orbitals. In a tetrahedral complex, the central metal ion requires four hybrid orbitals, which are formed by combining one d-orbital with three s and p orbitals.
The formation of coordination bonds in VBT is explained by the hybridization of the orbitals of the metal ion and the ligand, forming a new set of hybrid orbitals that overlap to form the coordinate covalent bond. The hybrid orbitals of the metal ion form sigma bonds with the ligand’s electron pairs. In VBT, the bonding in coordination compounds is explained by the overlap of the metal ion’s hybrid orbitals with the ligand’s lone pairs.
Crystal Field Theory (CFT)
Crystal Field Theory describes the bonding in coordination compounds as a result of the electrostatic interactions between the central metal ion and the ligands. According to CFT, the ligands form a crystal field around the metal ion that causes a splitting of the d-orbitals of the metal ion into two sets of energy levels: the t2g and eg sets.
The ligands in CFT are considered as point charges that create an electric field around the metal ion. The electric field affects the energies of the metal ion’s d-orbitals, causing the t2g orbitals to have a lower energy than the eg orbitals. The energy separation between the t2g and eg orbitals is known as the crystal field splitting energy (Δ). The presence of ligands results in a non-spherical electron distribution around the metal ion, which is called crystal field splitting.
In octahedral complexes, the ligands form an octahedral field around the central metal ion, which causes the d-orbitals to split into two sets: the t2g set and the eg set. In tetrahedral complexes, the ligands form a tetrahedral field around the central metal ion, which also causes the d-orbitals to split into two sets: the e set and the t2 set. The tetrahedral splitting energy is one-third of the octahedral splitting energy, as the tetrahedral field is less destabilizing than the octahedral field.
Conclusion
In conclusion, coordination compounds are essential molecules in chemistry, biochemistry, and materials science. The formation of coordination bonds is explained by Valence Bond Theory and Crystal Field Theory, which describe the bonding in coordination compounds as a result of the overlap of atomic or hybrid orbitals and the electrostatic interactions between the central metal ion and the ligands, respectively.
The coordination geometry of the complex determines the hybrid orbitals required for bonding, and the type of field around the central metal ion determines the splitting of the d-orbitals. In octahedral complexes, the d-orbitals split into the t2g and eg sets, while in tetrahedral complexes, the d-orbitals split into the e and t2 sets.
These theories allow us to understand the electronic structure and bonding in coordination compounds, which are essential in the design and development of materials and drugs, among other applications. The study of coordination compounds will continue to play a vital role in many areas of research and development.