
Chemical reactivity refers to the ability of a chemical substance to undergo a chemical reaction and to undergo a change in its chemical composition. It is determined by the arrangement of electrons in the atoms or molecules of a substance and their interactions with other atoms or molecules. Chemical reactivity is influenced by factors such as the type and strength of chemical bonds, the presence of functional groups, the size and shape of molecules, and the nature of the surrounding environment.
Chemical reactions can be classified into different types, such as oxidation-reduction reactions, acid-base reactions, and substitution reactions, based on the changes that occur in the chemical structure of the reactants and products. The rate and extent of a chemical reaction depend on various factors, such as temperature, pressure, concentration, and the presence of catalysts or inhibitors.
Understanding chemical reactivity is important in many fields, such as chemistry, biology, materials science, and environmental science. It can help us to design and optimize chemical processes, develop new materials with specific properties, and study the mechanisms of biological reactions and metabolic processes.
What is Required Chemical reactivity
“Required chemical reactivity” is not a commonly used term in chemistry. However, it could potentially refer to the desired or necessary level of reactivity that is needed for a particular chemical reaction to occur or for a specific chemical process to take place.
For example, in the field of organic synthesis, certain reactions may require a specific level of reactivity in order to proceed efficiently and selectively. This may be achieved by using particular reagents or catalysts that can promote the desired level of reactivity in the reaction mixture.
In the context of materials science, required chemical reactivity could refer to the ability of a material to undergo a certain chemical transformation or reaction, such as the ability of a catalyst to catalyze a specific chemical reaction.
Overall, the term “required chemical reactivity” would typically be used in a specific context or application, and its meaning would depend on that context.
Reactivity (chemistry)
In science, reactivity is the motivation for which a synthetic substance goes through a compound response, either without anyone else or with different materials, with a general arrival of energy.
Reactivity alludes to:
the compound responses of a solitary substance,
the synthetic responses of at least two substances that associate with one another,
the efficient investigation of sets of responses of these two sorts,
approach that applies to the investigation of reactivity of synthetics, everything being equal,
exploratory strategies that are utilized to notice these cycles
speculations to anticipate and to represent these cycles.
The synthetic reactivity of a solitary substance (reactant) covers its conduct where it:
Breaks down
Frames new substances by expansion of iotas from another reactant or reactants
Connects with at least two different reactants to shape at least two items
The synthetic reactivity of a substance can allude to the range of conditions (conditions that incorporate temperature, pressure, presence of impetuses) in which it responds, in blend with the:
Assortment of substances with which it responds
Harmony point of the response (i.e., the degree to which every last bit of it responds)
Pace of the response
The term reactivity is connected with the ideas of substance security and synthetic similarity.
Causes of reactivity
The second significance of ‘reactivity’, that of whether a substance responds, can be justified at the nuclear and sub-atomic level utilizing more seasoned and easier valence bond hypothesis and furthermore nuclear and sub-atomic orbital hypothesis. Thermodynamically, a synthetic response happens in light of the fact that the items (taken collectively) are at a lower free energy than the reactants; the lower energy state is alluded to as the ‘more steady state’. Quantum science gives the most top to bottom and correct comprehension of the explanation this happens. By and large, electrons exist in orbitals that are the aftereffect of addressing the Schrödinger condition for explicit circumstances.
All things (upsides of the n and ml quantum numbers) being equivalent, the request for soundness of electrons in a framework from least to most prominent is unpaired with no different electrons in comparative orbitals, unpaired with all savage orbitals half filled and the most steady is a filled arrangement of orbitals. To accomplish one of these sets of dependability, a molecule responds with one more iota to settle both. For instance, a solitary hydrogen molecule has a solitary electron in its 1s orbital. It turns out to be essentially more steady (as much as 100 kilocalories for every mole, or 420 kilojoules per mole) while responding to shape H2.
It is for this equivalent explanation that carbon quite often frames four bonds. Its ground state valence setup is 2s2 2p2, half filled. Notwithstanding, the actuation energy to go from half filled to completely filled p orbitals is so little it is irrelevant, and as such carbon structures them immediately. In the mean time, the cycle delivers a lot of energy (exothermic). This four equivalent bond setup is called sp3 hybridization.
The over three sections justify, but by and large, the responses of a few normal animal types, especially iotas. One way to deal with sum up the above is the enactment strain model of synthetic reactivity which gives a causal connection between, the reactants’ inflexibility and their electronic design, and the level of the response hindrance.
The pace of some random response,
is administered by the rate regulation:
where the rate is the adjustment of the molar fixation in one moment in the rate-deciding step of the response (the slowest step), [A] is the result of the molar convergence of the multitude of reactants raised to the right request, known as the response request, and k is the response consistent, which is steady for one given situation (by and large temperature and tension) and autonomous of focus. The more prominent the reactivity of a build the higher the worth of k and the higher the rate. For instance, if,
Then:
Rate=
where n is the reaction order of A, m is the reaction order of B, is the reaction order of the full reaction, and k is the reaction constant.
Chemical reaction
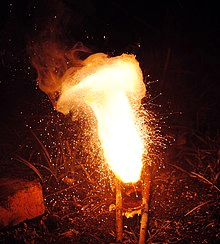
A compound response is a cycle that prompts the synthetic change of one bunch of compound substances to another. Traditionally, synthetic responses envelop changes that just include the places of electrons in the shaping and breaking of compound connections between molecules, with no change to the cores (no change to the components present), and can frequently be portrayed by a substance condition. Atomic science is a sub-discipline of science that includes the synthetic responses of shaky and radioactive components where both electronic and atomic changes can happen.
The substance (or substances) at first engaged with a compound response are called reactants or reagents. Substance responses are generally described by a synthetic change, and they yield at least one items, which typically have properties not the same as the reactants. Responses frequently comprise of a grouping of individual sub-steps, the supposed rudimentary responses, and the data on the exact strategy is important for the response system. Substance responses are depicted with synthetic conditions, which emblematically present the beginning materials, final results, and some of the time moderate items and response conditions.
Substance responses occur at a trademark response rate at a given temperature and compound focus. Normally, response rates increment with expanding temperature since there is more nuclear power accessible to arrive at the enactment energy essential for breaking connections between molecules. Responses might continue in the forward or switch bearing until they head to the end or arrive at harmony. Responses that continue in the forward bearing to move toward harmony are frequently depicted as unconstrained and lessen the free energy in the event that they happen at consistent temperature and strain. Non-unconstrained responses require a contribution of energy to proceed (models incorporate charging a battery driven by an outer electrical power source, or photosynthesis driven by retention of electromagnetic radiation typically as daylight).
A response might be named redox in which oxidation and decrease happen or nonredox in which there is no oxidation and decrease happening. Most straightforward redox responses might be named a blend, decay, or single removal response.
Different compound responses are utilized during substance union to acquire the ideal item. In organic chemistry, a continuous series of compound responses (where the result of one response is the reactant of the following response) structure metabolic pathways. These responses are frequently catalyzed by protein catalysts. Catalysts increment the paces of biochemical responses, so metabolic combinations and deteriorations inconceivable under standard circumstances can happen at the temperature and fixations present inside a cell.
The overall idea of a compound response has been stretched out to responses between substances less than iotas, including atomic responses, radioactive rots and responses between rudimentary particles, as depicted by quantum field hypothesis.
Reactivity (chemistry) 1
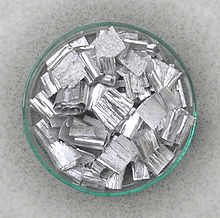
Reactivity is a proportion of how much a substance will in general respond with different things. A few substances are more receptive, and others are less responsive. Reactivity is set by a few variables:
the scope of conditions (conditions that incorporate temperature, tension, or presence of impetuses) in which the substance responds,
the scope of substances that will respond with it,
the balance point of the response (that is, the degree to which every last bit of it responds), or
the speed of the response.
Reactivity includes both the thermodynamics and energy of the specific compound response. A response will be more receptive in the event that the all out energy of the items is not exactly the all out energy of the reactants. A response will be more receptive on the off chance that the energy of its progress is low.
Lithium responds with water and air. Gold doesn’t respond with water or air. Gold is inert, consequently falling short on the reactivity series. Lithium is receptive. There are multiple ways of estimating reactivity. Some are lessening specialists, and others can be oxidizing specialists. Some ingest water without any problem. Some separate without any problem.
How is Required Chemical reactivity
“Required Chemical reactivity” is not a well-defined term in chemistry, so it is difficult to provide a precise answer to this question. However, in general, the level of chemical reactivity required for a particular chemical reaction or process can be influenced by a variety of factors, including the nature of the reactants and the reaction conditions.
For example, the choice of solvent, temperature, pressure, and the presence of catalysts or other additives can all impact the rate and extent of a chemical reaction. The reactivity of the reactants themselves, as determined by their electronic and steric properties, can also play a critical role in determining the outcome of a reaction.
In some cases, chemists may need to adjust the reactivity of one or more of the reactants in order to achieve the desired outcome. This could involve modifying the structure or functional groups of the reactants, or introducing catalysts or other additives to enhance reactivity.
Overall, the level of chemical reactivity required for a particular reaction or process is highly dependent on the specific context and goals of the experiment or application.
Case Study on Chemical reactivity
Here is a case study that illustrates the importance of chemical reactivity in the development of new materials.
Case Study: Developing More Efficient Solar Cells
The development of efficient and cost-effective solar cells is a major goal in renewable energy research. In recent years, researchers have been exploring the use of hybrid organic-inorganic perovskite materials as potential candidates for solar cell applications.
Perovskites are a class of materials with a unique crystal structure that exhibits excellent optical and electronic properties. However, perovskite solar cells suffer from stability issues and low efficiency, particularly in the long-term. To overcome these challenges, researchers have been exploring ways to modify the chemical structure of perovskite materials to enhance their performance and stability.
One approach to improving the stability of perovskite solar cells involves incorporating organic molecules into the crystal structure of the material. By doing so, researchers can modify the electronic and steric properties of the perovskite and tune its reactivity towards various chemical and physical stimuli.
For example, researchers at the University of California, Berkeley developed a new class of perovskite materials by incorporating bulky organic cations into the crystal structure of the material. The bulky cations acted as “molecular spacers” that prevented the perovskite structure from collapsing and improved its stability over time. The modified perovskite material also exhibited higher efficiency and improved thermal stability compared to traditional perovskite materials.
In another example, researchers at the University of Oxford modified the surface chemistry of perovskite materials by introducing functional groups that could act as anchors for other molecules. The modified perovskite was then used as a scaffold to attach various organic and inorganic molecules that could further enhance the properties of the material, such as its stability, optical absorption, or charge transport properties.
Overall, these examples illustrate how the reactivity of materials can be modified and tuned to improve their performance and stability in various applications. The development of new materials with tailored reactivity is an active area of research that has the potential to revolutionize many areas of science and technology, including energy conversion, catalysis, and materials science.
White paper on Chemical reactivity
Here is a brief white paper on Chemical Reactivity.
Introduction:
Chemical reactivity refers to the ability of a substance to undergo a chemical reaction. It is an essential concept in chemistry and plays a critical role in understanding how chemical reactions occur and how substances can be transformed into new compounds.
The level of reactivity of a substance can be influenced by a variety of factors, including the nature of the reactants, reaction conditions, and the presence of catalysts or other additives. In this white paper, we will explore the concept of chemical reactivity and its importance in various applications.
Key Concepts:
- Reactivity of Elements:
The reactivity of elements refers to their ability to form chemical bonds with other elements or compounds. The reactivity of an element is largely determined by its electron configuration and its position in the periodic table. For example, elements in group 1 of the periodic table, such as sodium and potassium, are highly reactive due to their tendency to lose an electron and form a positive ion.
- Factors Affecting Chemical Reactivity:
The reactivity of a substance can be influenced by a variety of factors, including temperature, pressure, and the presence of catalysts or other additives. Higher temperatures and pressures generally increase the rate of chemical reactions by increasing the energy of the reactant molecules. Catalysts can enhance the reactivity of substances by lowering the activation energy required for a reaction to occur.
- Applications of Chemical Reactivity:
The concept of chemical reactivity is important in many areas of chemistry and materials science. In organic chemistry, understanding the reactivity of different functional groups is critical for predicting the outcome of chemical reactions and developing new synthetic routes. In materials science, the reactivity of surfaces and interfaces plays a critical role in determining the properties of materials and their interactions with other substances.
- Safety Considerations:
Chemical reactivity can also have significant safety implications. Some substances, such as highly reactive metals or strong oxidizing agents, can react violently or explosively with other substances. Understanding the reactivity of substances and taking appropriate safety precautions is critical in many applications, such as chemical synthesis or handling hazardous materials.
Conclusion:
Chemical reactivity is a fundamental concept in chemistry that plays a critical role in many areas of science and technology. Understanding the reactivity of substances and how it can be modified or tuned is essential for developing new materials, predicting the outcomes of chemical reactions, and ensuring safety in chemical handling and processing. By continuing to study and explore the concept of chemical reactivity, we can unlock new possibilities and advancements in many fields.