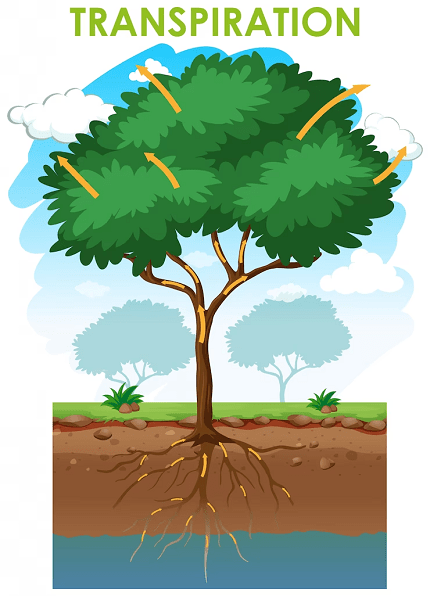
The effect of branching on alkanes, which are a type of hydrocarbon with only single bonds between carbon atoms, is to decrease their boiling points and increase their solubility in water.
When the carbon chain of an alkane is straight, the molecules can pack together more tightly, leading to stronger intermolecular forces and a higher boiling point. However, if there are branches on the carbon chain, the molecules can’t pack together as closely, which weakens the intermolecular forces and lowers the boiling point.
Additionally, branching increases the surface area of the molecule, which makes it easier for water molecules to surround and dissolve the molecule. This increases the solubility of the molecule in water.
Overall, the effect of branching on alkanes is to make them less volatile and more water-soluble compared to their straight-chain counterparts.
Branching (polymer chemistry)
In polymer science, spreading is the customary or unpredictable connection of side chains to a polymer’s spine chain. It happens by the substitution of a substituent (for example a hydrogen molecule) on a monomer subunit by another covalently-reinforced chain of that polymer; or, on account of a unite copolymer, by a chain of another kind. Spread polymers have more minimized and balanced atomic adaptations, and display intra-heterogeneous dynamical way of behaving as for the unbranched polymers. In crosslinking elastic by vulcanization, short sulfur branches connect polyisoprene chains (or an engineered variation) into a numerous extended thermosetting elastomer. Elastic can likewise be so totally vulcanized that it turns into an unbending strong, so hard it very well may be utilized as the piece in a smoking line. Polycarbonate chains can be crosslinked to shape the hardest, most effect safe thermosetting plastic, utilized in wellbeing glasses.
Spreading might result from the arrangement of carbon or different sorts of covalent bonds. Expanding by ester and amide bonds is regularly by a buildup response, delivering one particle of water (or HCl) for each bond shaped.
Polymers which are stretched however not crosslinked are for the most part thermoplastic. Spreading now and again happens unexpectedly during blend of polymers; e.g., by free-revolutionary polymerization of ethylene to shape polyethylene. Forestalling spreading to deliver straight polyethylene requires extraordinary strategies, as a matter of fact. Due to how polyamides are shaped, nylon would appear to be restricted to unbranched, straight chains. However, “star” expanded nylon can be created by the buildup of dicarboxylic acids with polyamines having at least three amino gatherings. Spreading likewise happens normally during enzymatically-catalyzed polymerization of glucose to frame polysaccharides like glycogen (creatures), and amylopectin, a type of starch (plants). The unbranched type of starch is called amylose.
A definitive in spreading is a totally crosslinked organization like tracked down in Bakelite, a phenol-formaldehyde thermoset pitch.
Branching factor
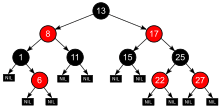
In processing, tree information designs, and game hypothesis, the stretching factor is the quantity of youngsters at every hub, the outdegree. On the off chance that this worth isn’t uniform, a normal spreading element can be determined.
For instance, in chess, if a “hub” is viewed as a lawful position, the typical expanding factor has been supposed to be around 35, and a measurable examination of over 2.5 million games uncovered a normal of 31. Overall, a player has around 31 to 35 lawful maneuvers available to them at each turn. By correlation, the typical expanding factor for the game Go is 250.
Higher stretching factors cause calculations that to follow each branch at each hub, for example, comprehensive beast force look, computationally more costly because of the dramatically expanding number of hubs, prompting combinatorial blast.
For instance, on the off chance that the fanning factor is 10, there will be 10 hubs one level down from the ongoing position, 102 (or 100) hubs two levels down, 103 (or 1,000) hubs three levels down, etc. The higher the fanning factor, the quicker this “blast” happens. The fanning element can be chopped somewhere near a pruning calculation.
The typical stretching variable can be immediately determined as the quantity of non-root hubs (the size of the tree, short one; or the quantity of edges) isolated by the quantity of non-leaf hubs (the quantity of hubs with kids).
Branching (linguistics)
In semantics, spreading alludes to the state of the parse trees that address the construction of sentences. Accepting that the language is being composed or interpreted from left to right, parse trees that develop down and to the right will be correct spreading, and parse trees that develop down and to the left will be left-expanding. The bearing of fanning mirrors the place of heads in phrases, and in such manner, right-spreading structures are head-beginning, though left-expanding structures are head-last. English has both right-expanding (head-introductory) and left-stretching (head-last) structures, in spite of the fact that it is more correct fanning than left-spreading. A few dialects, for example, Japanese and Turkish are completely left-spreading (head-last). A few dialects are generally correct expanding (head-beginning).
Branched flow
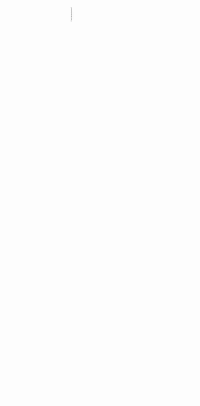
Spread stream alludes to a peculiarity in wave elements, that creates a tree-like example including progressive for the most part forward dispersing occasions by smooth hindrances redirecting voyaging beams or waves. Abrupt and huge force or wavevector changes are missing, however collected little changes can prompt enormous energy changes. The way of a solitary beam is less significant than the environs around a beam, which turn, pack, and stretch around in a space protecting way. Much more uncovering are gatherings, or manifolds of adjoining beams reaching out over critical zones. Beginning beams out from a point yet fluctuating their heading over a reach, one to the following, or from various focuses along a line all with similar introductory bearings are instances of a complex. Waves have undifferentiated from sending off conditions, for example, a point source showering in numerous bearings, or a lengthy plane wave heading on one course. The beam bowing or refraction prompts trademark structure in work space and nonuniform disseminations in coordinate space that look some way or another widespread and look like branches in trees or stream beds. The branches followed on non-clear ways through the refracting scene that are circuitous and nonlocal aftereffects of landscape previously navigated. For a given refracting scene, the branches will look totally changed relying upon the underlying complex.
Glycogen branching enzyme
Glycogen stretching protein is a catalyst that adds branches to the developing glycogen particle during the union of glycogen, a capacity type of glucose. All the more explicitly, during glycogen combination, a glucose 1-phosphate particle responds with uridine triphosphate (UTP) to become UDP-glucose, an enacted type of glucose. The enacted glucosyl unit of UDP-glucose is then moved to the hydroxyl bunch at the C-4 of a terminal buildup of glycogen to frame a α-1,4-glycosidic linkage, a response catalyzed by glycogen synthase. Critically, glycogen synthase can catalyze the amalgamation of α-1,4-glycosidic linkages. Since glycogen is a promptly prepared capacity type of glucose, the lengthy glycogen polymer is expanded by glycogen stretching chemical to give glycogen breakdown catalysts, for example, glycogen phosphorylase, with numerous terminal deposits for quick debasement. Spreading likewise critically builds the solvency and diminishes the osmotic strength of glycogen.
The protein encoded by this quality is a glycogen stretching catalyst that catalyzes the exchange of alpha-1,4-connected glucosyl units from the external finish of a glycogen chain to an alpha-1,6 situation on the equivalent or an adjoining glycogen chain. Expanding of the chains is fundamental for increment the dissolvability of the glycogen particle and, thus, in diminishing the osmotic tension inside cells. The most elevated levels of this protein are tracked down in liver and muscle cells. Transformations in this quality are related with glycogen capacity sickness type IV (otherwise called Andersen’s illness).
Case Study on Alkanes Effect of branching on them
Sure, here’s an example case study on the effect of branching on alkanes:
Case Study: Comparing the Properties of Branched and Straight-Chain Alkanes
Background: Alkanes are a class of hydrocarbons that consist of only carbon and hydrogen atoms, and they are widely used in various applications such as fuels, solvents, and lubricants. Alkanes can be classified into two main categories based on their carbon chain structure: straight-chain alkanes and branched alkanes. In this case study, we will compare the physical and chemical properties of two alkanes, one straight-chain and one branched, to investigate the effect of branching on their properties.
Materials and Methods: The two alkanes selected for this study are n-octane (a straight-chain alkane) and 2,2,4-trimethylpentane (a branched alkane). The properties that were compared include boiling point, density, viscosity, and flammability.
Results: Boiling Point: The boiling point of n-octane is 125°C, while the boiling point of 2,2,4-trimethylpentane is 101°C. The branched alkane has a lower boiling point than the straight-chain alkane due to the increased surface area of the branched molecule, which weakens the intermolecular forces and makes it easier to overcome them and reach the boiling point.
Density: The density of n-octane is 0.703 g/cm3, while the density of 2,2,4-trimethylpentane is 0.692 g/cm3. The branched alkane has a slightly lower density than the straight-chain alkane due to its more compact structure.
Viscosity: The viscosity of n-octane is 0.52 cP, while the viscosity of 2,2,4-trimethylpentane is 0.42 cP. The branched alkane has a lower viscosity than the straight-chain alkane due to its more spherical shape, which leads to less friction between the molecules.
Flammability: Both alkanes are highly flammable, but the branched alkane has a higher resistance to knocking (spontaneous combustion) than the straight-chain alkane due to its more compact and less reactive structure.
Conclusion: The results of this study demonstrate that the effect of branching on alkanes can significantly affect their physical and chemical properties. Branched alkanes tend to have lower boiling points, slightly lower densities, lower viscosities, and higher resistance to knocking than their straight-chain counterparts. These properties make branched alkanes suitable for use in certain applications, such as in gasoline additives, where high resistance to knocking is desirable.
White paper on Alkanes Effect of branching on them
Sure, here’s a white paper on the effect of branching on alkanes:
Title: The Effect of Branching on Alkanes: Physical and Chemical Properties
Introduction:
Alkanes are hydrocarbons with only single bonds between carbon atoms. They are widely used in various applications such as fuels, solvents, and lubricants. The physical and chemical properties of alkanes are largely dependent on their carbon chain structure. In this white paper, we will focus on the effect of branching on alkanes and its impact on their physical and chemical properties.
Effect of Branching on Boiling Point:
The boiling point of alkanes is largely dependent on the strength of intermolecular forces between their molecules. Straight-chain alkanes can pack more closely together due to their linear structure, leading to stronger intermolecular forces and higher boiling points. However, branched alkanes have a more compact and less linear structure, which leads to weaker intermolecular forces and lower boiling points. This effect is due to the increased surface area of the branched molecule, which reduces the ability of the molecules to pack together closely.
Effect of Branching on Density:
The density of alkanes is also dependent on their carbon chain structure. Branched alkanes have a more compact structure, which reduces the volume of space occupied by the molecule and leads to a slightly lower density compared to their straight-chain counterparts.
Effect of Branching on Viscosity:
The viscosity of alkanes is a measure of their resistance to flow. Branched alkanes have a more spherical shape, which leads to less friction between the molecules and a lower viscosity compared to their straight-chain counterparts.
Effect of Branching on Flammability:
The flammability of alkanes is largely dependent on their chemical structure. Branched alkanes have a more compact and less reactive structure, which leads to a higher resistance to knocking (spontaneous combustion) compared to their straight-chain counterparts.
Conclusion:
The effect of branching on alkanes can significantly affect their physical and chemical properties. Branched alkanes tend to have lower boiling points, slightly lower densities, lower viscosities, and higher resistance to knocking than their straight-chain counterparts. These properties make branched alkanes suitable for use in certain applications, such as in gasoline additives, where high resistance to knocking is desirable. Further research can be conducted to explore the impact of branching on the reactivity and other chemical properties of alkanes.