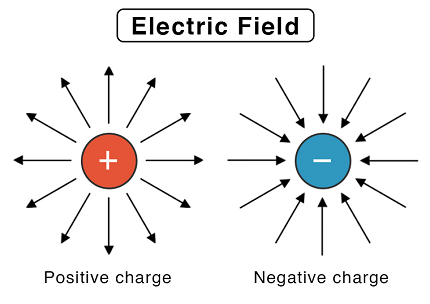
An electric field is a vector field that describes the influence exerted by electric charges on other charges in their vicinity. It is defined as the force per unit charge that a test charge would experience if placed in the field. Electric fields are produced by stationary charges, as well as by changing magnetic fields according to Maxwell’s equations.
Mathematically, the electric field E at any point in space is given by:
E = F/q
where F is the electric force experienced by a test charge q placed at that point, and E is the electric field vector. The direction of the electric field at a point is the same as the direction of the force that would be experienced by a positive test charge placed at that point.
The SI unit of electric field is Newtons per Coulomb (N/C), and it is commonly represented by the symbol E. Electric fields are fundamental to many areas of physics, including electromagnetism, electrostatics, and electrodynamics.
What is Required Electric field
The required electric field is the minimum electric field required to produce a desired effect or outcome. The specific value of the required electric field will depend on the context and application.
For example, in semiconductor device fabrication, a required electric field may be the minimum field necessary to cause electron-hole pairs to be generated in a photovoltaic cell, or the minimum field necessary to cause electrons to be injected into a transistor channel. In the context of particle accelerators, the required electric field may be the minimum field necessary to accelerate particles to a desired velocity or energy.
In general, the required electric field can be determined by analyzing the physical properties of the system and the desired outcome, and then applying relevant physical laws and equations to calculate the necessary field strength.
When is Required Electric field
The concept of required electric field arises in a wide range of applications in physics and engineering. In general, it is required whenever one needs to generate or control the motion of charged particles, or to induce a particular physical effect that is sensitive to the presence of an electric field.
Some specific examples of when the required electric field may arise include:
- In semiconductor device fabrication, the required electric field is necessary to induce carrier generation, injection, or drift, which are key processes for the operation of devices such as photovoltaic cells, transistors, and diodes.
- In plasma physics, the required electric field is used to ionize gases, generate plasma, and accelerate charged particles for fusion or propulsion applications.
- In high-energy physics, the required electric field is used to accelerate charged particles in particle accelerators, which are used to study fundamental particles and interactions.
- In electrochemistry, the required electric field is used to drive chemical reactions and electroplating processes.
- In medical imaging and therapy, the required electric field may be used to control the motion of charged particles for diagnostic or therapeutic purposes.
Overall, the required electric field is a key parameter for many applications in which electric charges play a central role, and it can be controlled by varying the strength, direction, and distribution of the electric field through appropriate design and control of the surrounding system.
Where is Required Electric field
The required electric field can be found in various physical systems and applications where electric charges are present, and it is used to induce a particular effect or outcome. Some common examples of where the required electric field can be found include:
- Semiconductor devices: In the fabrication of semiconductor devices such as transistors, diodes, and solar cells, a required electric field is needed to control the movement of electrons and holes in the material. This electric field is crucial for enabling the operation of the device.
- Electrochemical systems: In electrochemical systems, such as batteries and fuel cells, a required electric field is needed to drive the chemical reactions that produce or consume electric charge. The electric field can be applied externally or generated internally within the system.
- Plasma physics: In plasma physics, a required electric field is used to create and sustain a plasma, a state of matter in which ions and electrons are separated. The electric field is used to ionize the gas, and then to accelerate and control the motion of the charged particles.
- Particle accelerators: In particle accelerators, a required electric field is used to accelerate charged particles to high energies. The electric field is generated by a series of electrodes that produce a varying potential difference, which causes the particles to accelerate.
- Medical applications: In medical applications such as electroencephalography (EEG) and transcranial magnetic stimulation (TMS), a required electric field is used to stimulate the neurons in the brain. The electric field can be generated by applying a varying current to electrodes placed on the scalp.
Overall, the required electric field can be found in a wide range of physical systems and applications, and it is crucial for controlling the behavior of charged particles and inducing a desired effect or outcome.
How is Required Electric field
The required electric field can be determined by analyzing the physical properties of the system and the desired outcome, and then applying relevant physical laws and equations to calculate the necessary field strength.
The specific approach used to calculate the required electric field will depend on the system and the desired outcome. In general, one would need to consider the following factors:
- The charge distribution: The electric field depends on the charge distribution in the system. One needs to determine the positions and magnitudes of the charges in the system, and how they interact with each other.
- The geometry of the system: The geometry of the system can have a significant effect on the electric field. One needs to determine the shape and dimensions of the electrodes, conductors, or other components that generate or influence the electric field.
- The electric properties of the materials: The electric field also depends on the electric properties of the materials in the system, such as their dielectric constant, conductivity, and permittivity. These properties can affect how the electric field is distributed and propagated in the system.
- The physical laws governing the system: Finally, one needs to apply relevant physical laws and equations to calculate the electric field. For example, one may use Coulomb’s law to calculate the electric force between charges, or Poisson’s equation to calculate the electric potential distribution in a system.
Once these factors have been taken into account, one can calculate the required electric field by applying appropriate physical laws and equations. The specific value of the required electric field will depend on the context and application, and may need to be adjusted through experimental measurements and feedback control.
Nomenclature of Electric field
The electric field is a fundamental concept in physics that describes the force exerted on a charged particle at any given point in space. The electric field is represented by the symbol “E” and is defined as the force per unit charge experienced by a test charge placed at a given point.
The SI unit of electric field is the volt per meter (V/m), which represents the potential difference per unit length. Other common units of electric field include newtons per coulomb (N/C) and electric field units (e.u.).
The electric field can be classified into several types based on its source and characteristics, such as:
- Uniform Electric Field: This type of electric field has the same strength and direction at every point in space and is produced by two parallel plates with opposite charges.
- Radial Electric Field: This type of electric field has a spherical symmetry and is produced by a point charge.
- Non-Uniform Electric Field: This type of electric field has varying strength and direction at different points in space and is produced by any configuration of charges.
- Alternating Electric Field: This type of electric field changes direction and strength periodically with time and is produced by alternating current (AC).
- Static Electric Field: This type of electric field remains constant with time and is produced by a static charge.
Understanding the nomenclature and characteristics of electric fields is important in various fields such as electrical engineering, physics, and electronics.
Case Study on Electric field
Case Study: The Use of Electric Field in Electrostatic Precipitators
Electrostatic precipitators (ESP) are used in various industries to remove particulate matter (PM) from exhaust gases before they are released into the atmosphere. One of the main components of an ESP is an electric field, which is used to ionize the particles and then collect them on a grounded surface.
The basic principle of an ESP is to use an electric field to charge the particles in the exhaust gas. The particles are then attracted to a grounded plate or tube, where they are collected and removed. The electric field is typically generated by a series of high-voltage wires or plates, which are charged to several thousand volts.
The efficiency of an ESP depends on the strength and uniformity of the electric field. A uniform electric field ensures that all particles in the exhaust gas are charged to the same level, while a strong electric field ensures that the particles are attracted to the collection surface with sufficient force to overcome the drag and other forces that would otherwise prevent them from being collected.
The design and optimization of an ESP involves a careful balance between the strength and uniformity of the electric field, the flow rate and temperature of the exhaust gas, and the size and density of the particles. Computational fluid dynamics (CFD) simulations and experimental testing are used to determine the optimal configuration of the electric field and other components of the ESP.
The use of electric fields in ESPs has significant environmental benefits, as it allows for the removal of PM from exhaust gases before they are released into the atmosphere. This can reduce air pollution and improve air quality, particularly in areas with high levels of industrial activity or traffic.
In summary, the use of electric fields in ESPs is a practical application of the principles of electric fields in removing particulate matter from exhaust gases. The design and optimization of the electric field in an ESP involves a careful balance between various factors, and the use of computational simulations and experimental testing is necessary to achieve optimal performance.
White paper on Electric field
Electric Field: Understanding the Basics and Applications
Introduction:
Electric fields are fundamental to the behavior of electrically charged particles, such as electrons and protons, and they play a critical role in many areas of science and engineering. This white paper provides an overview of the basic principles of electric fields and their applications.
What is an Electric Field?
An electric field is a physical field that surrounds an electrically charged object and exerts a force on any other charged object placed within the field. Electric fields are described in terms of their strength and direction, and are typically represented by vectors.
The strength of an electric field at a given point in space is determined by the charge of the object that is creating the field and the distance from that object. The direction of the electric field is determined by the direction of the force that would be exerted on a positive test charge placed at that point.
Applications of Electric Fields:
Electric fields have a wide range of applications in science and engineering, including:
- Electrostatic Precipitators: As mentioned in the previous case study, electric fields are used in electrostatic precipitators to remove particulate matter from exhaust gases.
- Capacitors: Capacitors are electrical devices that store energy in an electric field between two charged plates. Capacitors are used in electronic circuits for energy storage, filtering, and coupling.
- Electric Motors: Electric motors use electric fields to convert electrical energy into mechanical energy. In a motor, the electric field produced by the current flowing through a wire interacts with the magnetic field produced by a magnet, causing the wire to rotate.
- Charged Particle Accelerators: Electric fields are used to accelerate charged particles, such as electrons or protons, to high speeds in particle accelerators. The electric field exerts a force on the charged particles, accelerating them along the length of the accelerator.
- Lightning Rods: Lightning rods are used to protect buildings and other structures from lightning strikes. They work by creating a strong electric field that attracts the lightning strike to the rod instead of the building.
Conclusion:
Electric fields are a fundamental concept in physics and have a wide range of applications in science and engineering. The strength and direction of an electric field are determined by the charge of the object creating the field and the distance from that object. Electric fields are used in electrostatic precipitators, capacitors, electric motors, charged particle accelerators, and lightning rods, among other applications. Understanding the principles and applications of electric fields is critical to many areas of modern technology and science.