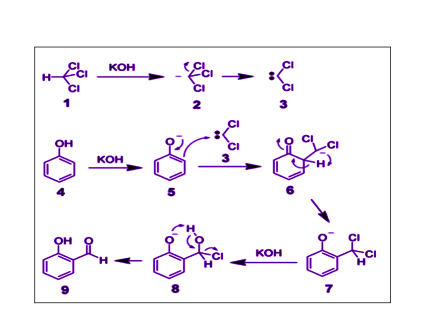
Phenol undergoes electrophilic substitution reactions due to the presence of the electron-rich aromatic ring and the electron-withdrawing effect of the hydroxyl group. Here are some examples of electrophilic substitution reactions of phenol:
- Nitration: When phenol is treated with a mixture of nitric and sulfuric acid, it undergoes nitration to form 2-nitrophenol. The nitronium ion is the electrophile in this reaction.
- Halogenation: Phenol undergoes halogenation when treated with a halogen (chlorine, bromine, or iodine) in the presence of a Lewis acid catalyst such as iron(III) chloride. The electrophile is the halonium ion generated in situ.
- Sulfonation: Phenol can be sulfonated with sulfuric acid to form phenol-2-sulfonic acid. Sulfur trioxide is the electrophile in this reaction.
- Friedel-Crafts acylation: Phenol undergoes acylation when treated with an acyl chloride in the presence of a Lewis acid catalyst such as aluminum chloride. The electrophile is the acylium ion generated in situ.
- Friedel-Crafts alkylation: Phenol undergoes alkylation when treated with an alkyl halide in the presence of a Lewis acid catalyst such as aluminum chloride. However, this reaction is not commonly used due to the tendency of the alkyl group to undergo further reactions leading to decomposition.
What is Required Electrophilic substitution reactions of phenol
For electrophilic substitution reactions of phenol to occur, the following conditions are required:
- Presence of an electrophile: An electrophile is a species that is attracted to electron-rich sites and has a positive or partial positive charge. In electrophilic substitution reactions of phenol, the electrophile can be a nitronium ion, halonium ion, sulfur trioxide, acylium ion, or carbocation.
- Presence of a Lewis acid catalyst: A Lewis acid catalyst such as aluminum chloride, iron(III) chloride, or zinc chloride is required to activate the electrophile and facilitate the reaction.
- Presence of a solvent: A solvent is required to dissolve the reactants and facilitate the reaction. Common solvents used in electrophilic substitution reactions of phenol include dichloromethane, chloroform, and nitrobenzene.
- Temperature: The temperature of the reaction mixture is an important factor that affects the rate of the reaction. Higher temperatures generally lead to faster reactions.
- Reaction time: The reaction time depends on the specific reaction conditions and the desired degree of substitution.
Overall, electrophilic substitution reactions of phenol require the presence of an electrophile, a Lewis acid catalyst, a solvent, appropriate reaction conditions, and appropriate reactants to produce the desired product.
When is Required Electrophilic substitution reactions of phenol
Electrophilic substitution reactions of phenol are useful in organic synthesis for the preparation of a variety of important compounds. Here are some examples:
- Preparation of nitrophenols: Nitrophenols are important intermediates in the synthesis of dyes, pharmaceuticals, and pesticides. Electrophilic nitration of phenol can be used to prepare various nitrophenols.
- Preparation of phenolic ethers: Phenolic ethers are important intermediates in the synthesis of pharmaceuticals, agrochemicals, and materials. Electrophilic substitution of phenol with an alkyl or aryl halide followed by deprotonation can be used to prepare phenolic ethers.
- Preparation of phenolic ketones: Phenolic ketones are important intermediates in the synthesis of pharmaceuticals, fragrances, and flavorings. Friedel-Crafts acylation of phenol can be used to prepare various phenolic ketones.
- Preparation of salicylic acid: Salicylic acid is an important compound used in the synthesis of aspirin and other pharmaceuticals. Electrophilic substitution of phenol with sulfur trioxide followed by hydrolysis can be used to prepare salicylic acid.
- Preparation of phenyl acetate: Phenyl acetate is an important compound used in the synthesis of fragrances and flavorings. Friedel-Crafts acylation of phenol with acetic anhydride can be used to prepare phenyl acetate.
Overall, electrophilic substitution reactions of phenol are used when the introduction of a new functional group onto the aromatic ring is required to prepare a specific compound or intermediate in organic synthesis.
Where is Required Electrophilic substitution reactions of phenol
Electrophilic substitution reactions of phenol can be carried out in a laboratory setting, in industrial processes, or in a combination of both.
In a laboratory setting, electrophilic substitution reactions of phenol can be carried out using standard glassware and equipment such as round-bottom flasks, reflux condensers, magnetic stirrers, and vacuum filtration apparatus. Small-scale reactions are typically carried out using a few grams of reactants and solvents, while larger-scale reactions may involve hundreds or even thousands of grams of reactants and solvents.
In industrial processes, electrophilic substitution reactions of phenol are typically carried out using specialized equipment designed for large-scale chemical production. These may include reactors, distillation columns, heat exchangers, and various types of pumps and valves. Industrial processes may also involve the use of automated control systems to monitor and optimize reaction conditions and to ensure consistent product quality.
Overall, the location of electrophilic substitution reactions of phenol depends on the specific application and scale of the reaction, with laboratory-scale reactions typically being carried out in research or academic settings, while industrial-scale reactions are typically carried out in specialized production facilities.
How is Required Electrophilic substitution reactions of phenol
The mechanism for electrophilic substitution reactions of phenol involves the attack of an electrophile on the electron-rich benzene ring of phenol. The steps involved in this reaction are as follows:
- Activation of the electrophile: The electrophile is usually activated by a Lewis acid catalyst such as aluminum chloride, iron(III) chloride, or zinc chloride. The Lewis acid catalyzes the formation of an electrophilic species, such as a nitronium ion, halonium ion, sulfur trioxide, acylium ion, or carbocation.
- Attack of the electrophile on the benzene ring: The electrophile attacks the electron-rich benzene ring of phenol, forming a resonance-stabilized intermediate. The intermediate has a positive charge on the carbon atom that is bonded to the electrophile and a negative charge on the oxygen atom of the phenol.
- Rearrangement of the intermediate: The intermediate undergoes a rearrangement, either by proton transfer or by a shift of the electron density, to form a more stable intermediate.
- Loss of a proton: The more stable intermediate then loses a proton from the oxygen atom to regenerate the aromatic ring.
- Regeneration of the catalyst: The catalyst is regenerated by reacting with the byproduct of the reaction, such as the halide ion, and is ready to catalyze the next round of electrophilic substitution.
The specific mechanism and intermediates involved in electrophilic substitution reactions of phenol depend on the nature of the electrophile and the reaction conditions. However, the basic steps outlined above are common to all electrophilic substitution reactions of phenol.
Nomenclature of Electrophilic substitution reactions of phenol
The nomenclature of electrophilic substitution reactions of phenol depends on the specific electrophile that is added to the benzene ring. Here are some examples:
- Nitration: When phenol is nitrated with nitric acid in the presence of sulfuric acid, the resulting compound is called nitrophenol. The position of the nitro group is indicated by a number, such as 2-nitrophenol or 4-nitrophenol.
- Halogenation: When phenol is halogenated with a halogen, such as chlorine or bromine, in the presence of a Lewis acid catalyst, the resulting compound is called a halophenol. The position of the halogen is indicated by a number, such as 2-chlorophenol or 4-bromophenol.
- Sulfonation: When phenol is sulfonated with sulfur trioxide, the resulting compound is called a phenol sulfonic acid. The position of the sulfonic acid group is indicated by a number, such as 2-phenol sulfonic acid or 4-phenol sulfonic acid.
- Friedel-Crafts acylation: When phenol is acylated with an acyl halide or an acid anhydride in the presence of a Lewis acid catalyst, the resulting compound is called a phenolic ketone. The position of the acyl group is indicated by a number, such as 2-acetylphenol or 4-benzoylphenol.
- Friedel-Crafts alkylation: When phenol is alkylated with an alkyl halide or an alkyl sulfate in the presence of a Lewis acid catalyst, the resulting compound is called a phenolic ether. The alkyl group is indicated by a prefix, such as methylphenol or ethylphenol.
Overall, the nomenclature of electrophilic substitution reactions of phenol follows standard organic chemistry nomenclature rules and depends on the nature and position of the substituent added to the benzene ring.
Case Study on Electrophilic substitution reactions of phenol
One example of a case study on electrophilic substitution reactions of phenol involves the synthesis of salicylic acid, a common precursor to aspirin. The synthesis of salicylic acid from phenol involves a series of electrophilic substitution reactions.
First, phenol is nitrated with a mixture of nitric and sulfuric acid to yield 2-nitrophenol. The position of the nitro group is controlled by the electronic properties of the phenol ring, with the nitro group preferentially adding to the ortho position relative to the hydroxyl group. The resulting 2-nitrophenol is then reduced with a reducing agent, such as iron or zinc, to yield 2-aminophenol.
The amino group is then diazotized with nitrous acid to yield a diazonium salt. The diazonium salt is then coupled with sodium salicylate, which is prepared from salicylic acid and sodium hydroxide, to yield salicylic acid. The coupling reaction involves the electrophilic substitution of the diazonium ion onto the aromatic ring of the salicylate, followed by elimination of nitrogen gas to yield the substituted salicylic acid product.
This case study demonstrates the versatility of electrophilic substitution reactions of phenol and their ability to generate a wide range of substituted products. The specific reactions and mechanisms involved in this synthesis also highlight the importance of careful control of reaction conditions and the use of specialized reagents and techniques, such as diazotization and coupling reactions, in organic synthesis.
White paper on Electrophilic substitution reactions of phenol
Introduction Electrophilic substitution reactions of phenol are a key area of study in organic chemistry. Phenol, a cyclic organic compound consisting of a benzene ring with a hydroxyl group attached, is highly reactive due to the presence of a π electron system in the ring. As a result, it readily undergoes electrophilic substitution reactions, in which an electrophile is added to the benzene ring, replacing a hydrogen atom. In this white paper, we will explore the mechanisms and applications of electrophilic substitution reactions of phenol.
Mechanisms of Electrophilic Substitution Reactions of Phenol Electrophilic substitution reactions of phenol are typically classified into two categories: reactions that occur with strong electrophiles and reactions that occur with weak electrophiles.
Reactions with Strong Electrophiles: In these reactions, strong electrophiles, such as nitric acid or sulfuric acid, are added to the phenol ring. The hydroxyl group in phenol acts as a weak base, and it can donate electrons to the ring, making it more electron-rich and more susceptible to attack by electrophiles. The reaction mechanism typically involves the formation of an intermediate complex, followed by the loss of a proton to regenerate the aromaticity of the benzene ring. For example, in the nitration of phenol, the reaction mechanism involves the formation of a nitronium ion intermediate, which then adds to the ring to form a substituted nitrophenol product.
Reactions with Weak Electrophiles: In these reactions, weak electrophiles, such as halogens or alkyl halides, are added to the phenol ring. The reaction mechanism involves the formation of a carbocation intermediate, which then reacts with the phenol ring to form a substituted product. In some cases, a Lewis acid catalyst is used to facilitate the formation of the carbocation intermediate. For example, in the bromination of phenol, the reaction mechanism involves the formation of a bromonium ion intermediate, which then adds to the ring to form a substituted bromophenol product.
Applications of Electrophilic Substitution Reactions of Phenol Electrophilic substitution reactions of phenol have numerous applications in organic synthesis. Some common examples include:
- Synthesis of Salicylic Acid: Salicylic acid, a precursor to aspirin, is synthesized via a series of electrophilic substitution reactions. Nitration of phenol yields 2-nitrophenol, which can be reduced to yield 2-aminophenol. The amino group is then diazotized and coupled with sodium salicylate to yield salicylic acid.
- Synthesis of Phenolic Ethers: Phenolic ethers, which have a wide range of industrial applications, can be synthesized via electrophilic substitution reactions of phenol with alkyl halides or alkyl sulfates in the presence of a Lewis acid catalyst.
- Synthesis of Phenol Sulfonic Acids: Phenol sulfonic acids, which are used as surfactants and in the production of dyes and pigments, can be synthesized via electrophilic substitution reactions of phenol with sulfur trioxide.
Conclusion
In conclusion, electrophilic substitution reactions of phenol are an important topic in organic chemistry, which allow the synthesis of a wide range of substituted products. These reactions are highly versatile and can be used for various applications in pharmaceuticals, dyes, and surfactants. The reaction mechanisms are complex, and involve the formation of intermediate species, which require careful control to achieve specific outcomes. Therefore, a deeper understanding of the mechanisms and applications of electrophilic substitution reactions of phenol can help researchers to design and optimize new synthetic routes, and ultimately, advance the field of organic chemistry.