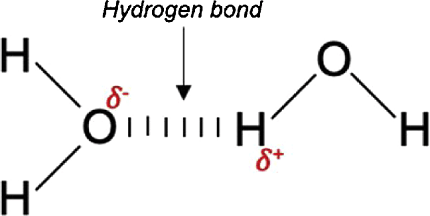
A hydrogen bond is a type of intermolecular force that occurs between a hydrogen atom bonded to an electronegative atom, such as nitrogen, oxygen, or fluorine, and another electronegative atom. The hydrogen atom has a partial positive charge due to the electron-withdrawing effect of the more electronegative atom, while the other atom has a partial negative charge. This results in an electrostatic attraction between the two atoms, which is known as a hydrogen bond.
Hydrogen bonds are important in many biological processes, including the structure and stability of DNA and proteins. They also play a critical role in the properties of water, as they help to hold water molecules together and give water its high boiling point and surface tension. In addition, hydrogen bonds are responsible for many of the unique properties of other compounds, such as the solubility of alcohols and the high melting and boiling points of some organic molecules.
Carbon–hydrogen bond
In science, the carbon-hydrogen bond (C−H bond) is a substance connection among carbon and hydrogen iotas that can be tracked down in numerous natural mixtures. This bond is a covalent, single bond, implying that carbon imparts its external valence electrons to up to four hydrogens. This finishes both of their external shells, making them stable.
Carbon-hydrogen bonds have a bond length of around 1.09 Å (1.09 × 10−10 m) and a bond energy of around 413 kJ/mol (see table underneath). Utilizing Pauling’s scale — C (2.55) and H (2.2) — the electronegativity distinction between these two particles is 0.35. In light of this little distinction in electronegativities, the C−H bond is by and large viewed as being non-polar. In underlying recipes of particles, the hydrogen iotas are frequently overlooked. Compound classes comprising exclusively of C−H bonds and C−C bonds are alkanes, alkenes, alkynes, and sweet-smelling hydrocarbons. On the whole they are known as hydrocarbons.
In October 2016, cosmologists detailed that the extremely essential substance elements of life — the carbon-hydrogen atom (CH, or methylidyne revolutionary), the carbon-hydrogen positive particle (CH+) and the carbon particle (C+) — are the outcome, to a great extent, of bright light from stars, as opposed to in alternate ways, for example, the consequence of violent occasions connected with supernovae and youthful stars, as thought prior.
Hydrogen-bond catalysis

Hydrogen-security catalysis is a kind of organocatalysis that depends on utilization of hydrogen holding collaborations to speed up and control natural responses. In organic frameworks, hydrogen holding assumes a vital part in numerous enzymatic responses, both in situating the substrate particles and bringing obstructions down to response. In any case, scientific experts have as of late endeavored to tackle the force of utilizing hydrogen bonds to perform catalysis, and the field is somewhat lacking contrasted with research in Lewis corrosive catalysis.
Synergist measures of hydrogen-bond givers can advance responses through a wide range of components. Over the span of a response, hydrogen holding can be utilized to settle anionic intermediates and progress states. On the other hand, a few impetuses can tie little anions, empowering the development of responsive electrophilic cations. More acidic benefactors can go about as broad or explicit acids, which enact electrophiles by protonation. A strong methodology is the concurrent enactment of the two accomplices in a response, for example nucleophile and electrophile, named “bifunctional catalysis”. In all cases, the nearby relationship of the impetus particle to substrate likewise makes hydrogen-security catalysis a strong strategy for prompting enantioselectivity.
Hydrogen-holding impetuses are many times easy to make, generally hearty, and can be combined in high enantiomeric virtue. New responses catalyzed by hydrogen-bond contributors are being found at a rising speed, including topsy-turvy variations of normal natural responses helpful for amalgamation, like aldol augmentations, Diels-Birch cycloadditions and Mannich responses.
In any case, there are a few difficulties that should be defeated before hydrogen-bond catalysis can accomplish its maximum capacity with regards to engineered utility. Current realized responses are very substrate explicit and for the most part show low rate speed increase and turnover, hence requiring high impetus stacking. Impetuses are frequently found and streamlined by experimentation, and scientific experts have an unfortunate comprehension of the connection between impetus construction and reactivity. Furthermore, the field experiences an absence of general unthinking comprehension, which has been enormously dominated by the disclosure of new responses. With additional point by point investigations of construction and system later on, hydrogen-bond catalysis has extraordinary potential for empowering new, productive, specific responses and promising applications in unbalanced amalgamation.
Chemical bond
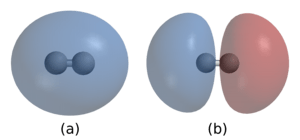
A substance bond is an enduring fascination between iotas or particles that empowers the development of atoms and gems. The bond might result from the electrostatic power between oppositely charged particles as in ionic bonds, or through the sharing of electrons as in covalent bonds. The strength of compound bonds changes significantly; there are “solid bonds” or “essential bonds” like covalent, ionic and metallic bonds, and “feeble bonds” or “optional bonds, for example, dipole collaborations, the London scattering power, and hydrogen holding. Solid substance holding emerges from the sharing or move of electrons between the taking part molecules.
Since inverse electric charges draw in, the adversely charged electrons encompassing the core and the emphatically charged protons inside a core draw in one another. An electron situated between two cores will be drawn to the two of them, and the cores will be drawn in toward electrons here. This fascination is a covalent compound bond. In view of the matter wave nature of electrons and their more modest mass, they possess a lot bigger volume than the cores, and this volume keeps the nuclear cores in a bond far separated contrasted with the size of the cores.
The particles in atoms, precious stones, metals and a large portion of the actual climate around and inside us are kept intact by substance bonds, which decide the design and properties of issue.
All bonds can be made sense of by quantum hypothesis, however, by and by, worked on rules and speculations permit scientists to anticipate the strength, directionality, and extremity of bonds. The octet rule and VSEPR hypothesis are models. More modern hypotheses are valence bond hypothesis, which incorporates orbital hybridization and reverberation, and sub-atomic orbital hypothesis which incorporates the straight blend of nuclear orbitals and ligand field hypothesis. Electrostatics are utilized to portray bond polarities and the impacts they have on synthetic substances.
Hydrogen-bonded organic framework
Hydrogen-fortified natural systems (HOFs) are a class of a few layered materials shaped by hydrogen bonds among sub-atomic monomer units to manage the cost of porosity and underlying adaptability (Fig. 1). There are assorted hydrogen holding pair decisions that could be utilized in HOFs development, including indistinguishable or nonidentical hydrogen holding givers and acceptors. For natural gatherings going about as hydrogen holding units, species like carboxylic corrosive, amide, 2,4-diaminotriazine, and imidazole, and so on, are ordinarily utilized for the arrangement of hydrogen holding communication. Contrasted and other natural systems, as COF and MOF, the limiting power of HOFs is somewhat more vulnerable and the initiation of HOFs is more troublesome than different structures, while the reversibility of hydrogen bonds ensures a high crystallinity of the materials. However the strength and pore size extension of HOFs has possible issues, HOFs actually show solid potential for applications in various regions.
Significant outcome of the normal permeable design of hydrogen-reinforced natural structures is to understand the adsorption of visitor particles. This character speeds up the development of different uses of various HOFs structures, including gas expulsion/capacity/division, particle acknowledgment, proton conduction, and biomedical applications, and so on.
Low-barrier hydrogen bond
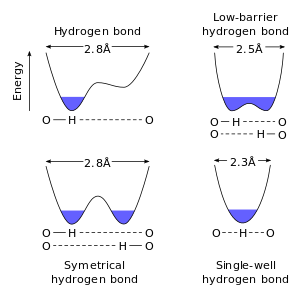
A Low-hindrance hydrogen bond (LBHB) is a unique kind of hydrogen bond. LBHBs can happen when the pKa of the two heteroatoms are firmly coordinated, which permits the hydrogen to be all the more similarly divided among them. This hydrogen-sharing causes the development of particularly short, solid hydrogen bonds.
Case Study on Hydrogen bond
Sure, I can provide you with an example of a case study on hydrogen bonds.
One example of the importance of hydrogen bonds in biological systems is the structure of DNA. The double helix structure of DNA is held together primarily by hydrogen bonds between the complementary base pairs of adenine (A) and thymine (T), as well as between the complementary base pairs of guanine (G) and cytosine (C).
Each base pair consists of a purine base (adenine or guanine) and a pyrimidine base (thymine or cytosine). The purine and pyrimidine bases are held together by hydrogen bonds, with three hydrogen bonds between G and C, and two hydrogen bonds between A and T. These hydrogen bonds contribute significantly to the stability of the DNA molecule, as they help to hold the two strands of the double helix together.
Furthermore, the specific pairing of A-T and G-C base pairs is essential for the accurate replication and transmission of genetic information from one generation to the next. Any disruption in the hydrogen bonding between base pairs can lead to errors in DNA replication or transcription, which can have serious consequences for the organism.
In addition to DNA, hydrogen bonds are also important in many other biological processes, including protein folding, enzyme-substrate interactions, and the binding of small molecules such as drugs to their targets. Understanding the role and importance of hydrogen bonds in these processes can help to inform the design of new drugs and therapies, as well as advance our understanding of fundamental biological processes.
White paper on Hydrogen bond
Here is an overview of a white paper on hydrogen bonds:
Title: The Importance of Hydrogen Bonds in Chemistry and Biology
Abstract:
Hydrogen bonds are a fundamental type of intermolecular interaction that play a critical role in many chemical and biological processes. In this white paper, we explore the nature and properties of hydrogen bonds, their importance in chemistry and biology, and the various applications of hydrogen bonding in different fields.
Introduction:
The concept of hydrogen bonding was first introduced in the early 20th century, and has since become an essential part of our understanding of chemical and biological systems. Hydrogen bonds arise from the interaction between a hydrogen atom and a highly electronegative atom, such as nitrogen, oxygen, or fluorine. These bonds are much weaker than covalent bonds, but are still strong enough to influence the structure and properties of molecules and materials.
Properties and Nature of Hydrogen Bonds:
In this section, we discuss the unique properties of hydrogen bonds, including their strength, directionality, and specificity. We also examine the different types of hydrogen bonds, such as intermolecular and intramolecular hydrogen bonds, and the factors that affect their strength and stability.
Importance of Hydrogen Bonds in Chemistry:
Hydrogen bonds have numerous applications in chemistry, ranging from the design of new materials to the development of new drugs and catalysts. We explore some of these applications, including the use of hydrogen bonding in supramolecular chemistry, crystal engineering, and molecular recognition.
Importance of Hydrogen Bonds in Biology:
Hydrogen bonds are essential for many biological processes, including protein folding, DNA replication and transcription, and enzyme-substrate interactions. We discuss the role of hydrogen bonds in these processes, and how disruptions in hydrogen bonding can lead to disease and dysfunction.
Applications of Hydrogen Bonding:
Finally, we examine the various applications of hydrogen bonding in different fields, such as materials science, pharmacology, and nanotechnology. We also explore the potential future applications of hydrogen bonding in areas such as energy storage, water purification, and drug delivery.
Conclusion:
In conclusion, hydrogen bonds are a critical part of our understanding of chemical and biological systems, with numerous applications in various fields. Further research into the nature and properties of hydrogen bonding will continue to advance our understanding of fundamental processes and lead to new applications and technologies.