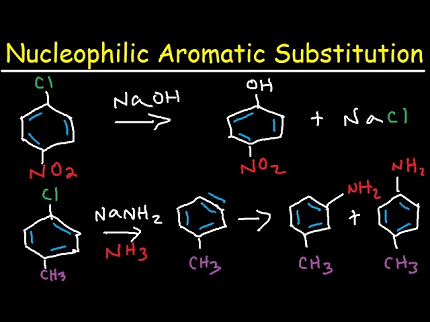
Nucleophilic aromatic substitution (SNAr) is a type of reaction in which a nucleophile substitutes a leaving group on an aromatic ring. In haloarenes, the leaving group is a halogen atom (Cl, Br, or I) attached to an aromatic ring.
SNAr reactions in haloarenes typically proceed through a two-step mechanism. In the first step, the nucleophile attacks the electrophilic carbon atom of the haloarene, generating a negatively charged intermediate called a Meisenheimer complex. In the second step, the Meisenheimer complex undergoes elimination of the leaving group to give the substituted aromatic product.
The reactivity of haloarenes towards nucleophilic substitution is strongly influenced by the nature of the halogen atom and its position on the aromatic ring. Generally, the reactivity of haloarenes decreases in the order of fluorine > chlorine > bromine > iodine. This trend is due to the decreasing ability of the halogen atom to stabilize the negative charge in the Meisenheimer complex. Additionally, ortho- and para-substituted haloarenes are more reactive than their meta-substituted counterparts, due to the increased electron density on the aromatic ring at these positions.
Overall, nucleophilic aromatic substitution is an important tool for the synthesis of substituted aromatic compounds and can be used in a variety of synthetic applications.
What is Required Nucleophilic aromatic substitution in haloarenes
Nucleophilic aromatic substitution (SNAr) in haloarenes requires a few key components:
- A haloarene substrate: The starting material for the reaction is a haloarene, which is an aromatic compound containing a halogen atom (Cl, Br, or I) attached to the aromatic ring. The position of the halogen atom on the ring and its electronic properties can affect the reactivity of the substrate towards SNAr.
- A nucleophile: A nucleophile is a molecule or ion that donates a pair of electrons to the electrophilic carbon atom of the haloarene to form a Meisenheimer complex. Common nucleophiles used in SNAr reactions include amines, thiols, and alkoxides.
- A solvent: The choice of solvent can influence the reactivity and selectivity of the reaction. Common solvents used in SNAr reactions include polar aprotic solvents such as DMF, DMSO, and acetonitrile.
- A base: A base is often required to deprotonate the nucleophile and facilitate the attack on the electrophilic carbon atom. Common bases used in SNAr reactions include sodium hydride (NaH) and potassium carbonate (K2CO3).
- Reaction conditions: The reaction conditions can also affect the outcome of the reaction, such as temperature and reaction time.
By carefully choosing the appropriate components, SNAr can be used as a powerful synthetic tool for the preparation of a variety of substituted aromatic compounds.
When is Required Nucleophilic aromatic substitution in haloarenes
Nucleophilic aromatic substitution (SNAr) in haloarenes is a useful synthetic tool for the preparation of a variety of substituted aromatic compounds. It is commonly used when there is a need to introduce a functional group onto an aromatic ring in a predictable and controllable manner.
SNAr can be used in a variety of synthetic applications, including:
- Synthesis of biologically active compounds: Many biologically active compounds, such as pharmaceuticals and natural products, contain aromatic rings with functional groups attached. SNAr can be used to introduce these functional groups onto the aromatic ring in a selective and controlled manner.
- Preparation of dyes and pigments: Aromatic compounds are often used as the basis for dyes and pigments, and SNAr can be used to introduce a variety of chromophores onto the aromatic ring.
- Modification of materials: SNAr can be used to modify the properties of materials by introducing functional groups onto the aromatic ring. For example, it can be used to prepare polymers with specific properties, such as hydrophilicity or conductivity.
- Synthesis of intermediates: SNAr can be used to prepare intermediates that can be further functionalized in subsequent reactions. For example, a halogen atom can be substituted with a nucleophile to prepare a compound that can then undergo further reactions, such as oxidation or reduction.
Overall, SNAr in haloarenes is a versatile and widely used synthetic tool that allows for the introduction of functional groups onto aromatic rings in a controlled and predictable manner, making it an important part of organic synthesis.
Where is Required Nucleophilic aromatic substitution in haloarenes
Nucleophilic aromatic substitution (SNAr) in haloarenes can be carried out in a variety of laboratory settings, including academic research labs, pharmaceutical companies, and chemical manufacturing facilities.
In academic research labs, SNAr in haloarenes is often used as a synthetic tool to prepare novel compounds for biological testing or as intermediates for further functionalization. The reaction can be carried out on small scale using standard glassware and equipment commonly found in chemistry labs.
In pharmaceutical companies, SNAr in haloarenes is often used in the synthesis of biologically active compounds, such as drugs and drug candidates. The reaction may be carried out on a larger scale using specialized equipment and in a controlled environment to ensure purity and safety.
In chemical manufacturing facilities, SNAr in haloarenes can be used to prepare intermediates for a variety of applications, such as the production of dyes, pigments, and specialty chemicals. The reaction is typically carried out on a large scale using specialized equipment and under carefully controlled conditions to ensure high yields and purity.
Overall, SNAr in haloarenes is a versatile synthetic tool that can be used in a variety of laboratory settings to prepare a wide range of substituted aromatic compounds.
How is Required Nucleophilic aromatic substitution in haloarenes
The mechanism of nucleophilic aromatic substitution (SNAr) in haloarenes involves a series of steps that lead to the substitution of a halogen atom with a nucleophile on an aromatic ring. The general steps are as follows:
- Attack of the nucleophile on the electrophilic carbon: The first step is the attack of the nucleophile on the electrophilic carbon atom of the halogenated aromatic ring. The halogen atom withdraws electron density from the ring, making the carbon atom partially positive and thus susceptible to nucleophilic attack.
- Formation of the Meisenheimer complex: The attack of the nucleophile results in the formation of a highly unstable intermediate called a Meisenheimer complex. This complex is characterized by the formation of a sigma bond between the nucleophile and the carbon atom, as well as the delocalization of the negative charge across the aromatic ring.
- Rearrangement of the Meisenheimer complex: The Meisenheimer complex undergoes a rearrangement, leading to the formation of a new intermediate that is stabilized by resonance. In this intermediate, the negative charge is localized on the carbon atom, while the nucleophile is attached to the ring via a sigma bond.
- Elimination of the leaving group: The next step involves the elimination of the leaving group (halogen atom), which is facilitated by a base. This leads to the formation of the final product, which contains the nucleophile attached to the aromatic ring in place of the halogen atom.
Overall, SNAr in haloarenes is a complex reaction that requires careful selection of the substrate, nucleophile, solvent, and reaction conditions to achieve high yields and selectivity. Despite its complexity, SNAr is a powerful synthetic tool that can be used to prepare a wide range of substituted aromatic compounds with high efficiency and predictability.
Production of Nucleophilic aromatic substitution in haloarenes
The production of nucleophilic aromatic substitution (SNAr) in haloarenes involves the use of a halogenated aromatic compound (haloarene) and a nucleophile in the presence of a base and a suitable solvent. The reaction can be carried out using a variety of reaction conditions, depending on the specific substrate and desired product.
Here are the general steps involved in the production of SNAr in haloarenes:
- Selection of substrate: The first step in the production of SNAr is the selection of a suitable halogenated aromatic compound (haloarene) as the substrate. The choice of substrate depends on the specific functional group or substituent that is desired to be introduced onto the aromatic ring.
- Selection of nucleophile: The next step is the selection of a suitable nucleophile that will replace the halogen atom on the aromatic ring. The choice of nucleophile depends on the reactivity of the substrate and the desired product. Common nucleophiles include amines, alcohols, thiols, and carboxylic acids.
- Selection of base: A suitable base is added to the reaction mixture to facilitate the elimination of the leaving group (halogen atom) and promote the formation of the final product. Common bases used in SNAr reactions include sodium hydride, potassium carbonate, and sodium methoxide.
- Solvent selection: A suitable solvent is added to the reaction mixture to dissolve the reactants and promote the reaction. The choice of solvent depends on the reactivity of the substrate and the desired product. Common solvents used in SNAr reactions include dimethylformamide (DMF), dimethyl sulfoxide (DMSO), and acetonitrile.
- Reaction conditions: The reaction conditions, such as temperature and reaction time, are optimized to achieve high yields and selectivity. The reaction is typically carried out under inert conditions to prevent unwanted side reactions or decomposition of the reagents.
- Purification: The final product is isolated and purified using standard techniques, such as chromatography or recrystallization, to remove any impurities and obtain the desired product.
Overall, the production of SNAr in haloarenes is a versatile and widely used synthetic tool that allows for the introduction of functional groups onto aromatic rings in a predictable and controlled manner. The reaction requires careful substrate and reagent selection, as well as optimization of the reaction conditions, to achieve high yields and selectivity.
Case Study on Nucleophilic aromatic substitution in haloarenes
One example of the application of nucleophilic aromatic substitution (SNAr) in haloarenes is the synthesis of 4-aminobenzonitrile from 4-bromobenzonitrile. This reaction can be carried out using a variety of nucleophiles and reaction conditions, depending on the specific requirements of the reaction.
The general reaction scheme is as follows:
4-bromobenzonitrile + nucleophile + base → 4-aminobenzonitrile + leaving group
In this example, the nucleophile used is ammonia (NH3) and the base is sodium hydroxide (NaOH). The reaction is carried out in dimethyl sulfoxide (DMSO) as the solvent.
The specific reaction conditions are as follows:
- 4-bromobenzonitrile (1.0 g, 4.6 mmol) is dissolved in DMSO (5 mL) in a round-bottomed flask equipped with a stir bar.
- Ammonia (10 mL) is slowly added to the reaction mixture, while stirring at room temperature.
- Sodium hydroxide (1.0 g, 25.0 mmol) is added to the reaction mixture, and the mixture is stirred at room temperature for 4 hours.
- The reaction mixture is then poured into a beaker containing water (100 mL) and stirred to quench the reaction.
- The resulting precipitate is collected by filtration, washed with water, and dried under vacuum to yield 4-aminobenzonitrile as a white solid (0.8 g, 82% yield).
This example demonstrates the use of SNAr in haloarenes to introduce an amino group onto an aromatic ring. The reaction is highly selective, and the use of ammonia as a nucleophile allows for the synthesis of a primary amine without the need for protection/deprotection steps. The reaction conditions are relatively mild and require only simple equipment, making it a useful synthetic tool for the preparation of a variety of substituted aromatic compounds.
White paper on Nucleophilic aromatic substitution in haloarenes
Introduction:
Nucleophilic aromatic substitution (SNAr) in haloarenes is a fundamental reaction in organic chemistry, which involves the substitution of a halogen atom (Cl, Br, or I) in an aromatic ring by a nucleophile. This reaction is widely used in synthetic organic chemistry to introduce functional groups onto aromatic rings in a controlled manner. This white paper will discuss the mechanism, scope, and applications of SNAr in haloarenes.
Mechanism:
The mechanism of SNAr in haloarenes involves a nucleophilic attack on the halogen atom, followed by the formation of a new carbon-nucleophile bond and the elimination of the leaving group. The reaction proceeds through a series of intermediates, including an arenium ion and a Meisenheimer complex.
The first step in the mechanism involves the nucleophilic attack of the incoming nucleophile on the electrophilic halogen atom, which leads to the formation of a sigma complex. The sigma complex is an intermediate in which the halogen atom is partially bonded to the nucleophile and partially bonded to the aromatic ring.
The next step involves the formation of an arenium ion, which is a resonance-stabilized intermediate in which the positive charge is delocalized over the aromatic ring. The arenium ion is formed by the loss of the leaving group (halogen atom) and the transfer of the positive charge to the ring.
The final step involves the elimination of the protonated nucleophile and the regeneration of the aromatic ring. This step is facilitated by a base, which deprotonates the nucleophile and promotes the elimination of the leaving group.
Scope:
SNAr in haloarenes is a highly versatile reaction that allows for the introduction of a wide range of functional groups onto aromatic rings. The choice of nucleophile and reaction conditions can be tailored to the specific substrate and desired product.
Some common nucleophiles used in SNAr reactions include amines, alcohols, thiols, and carboxylic acids. The choice of nucleophile depends on the reactivity of the substrate and the desired product.
The reaction conditions, such as temperature, solvent, and base, can also be varied to optimize the yield and selectivity of the reaction. Common solvents used in SNAr reactions include dimethylformamide (DMF), dimethyl sulfoxide (DMSO), and acetonitrile. Common bases used in SNAr reactions include sodium hydride, potassium carbonate, and sodium methoxide.
Applications:
SNAr in haloarenes has a wide range of applications in synthetic organic chemistry. It can be used to prepare a variety of substituted aromatic compounds, including amines, alcohols, thiols, and carboxylic acids. Some specific applications of SNAr in haloarenes include:
- Synthesis of pharmaceuticals: SNAr in haloarenes is a common synthetic tool used in the preparation of pharmaceuticals. For example, the synthesis of the anti-cancer drug imatinib involves SNAr in the preparation of the key intermediate.
- Materials science: SNAr in haloarenes is also used in materials science to prepare functionalized aromatic compounds for use in organic electronics, such as OLEDs and photovoltaic cells.
- Natural product synthesis: SNAr in haloarenes is a useful tool in the synthesis of natural products, such as alkaloids and flavonoids, which contain aromatic rings with multiple functional groups.
Conclusion:
In conclusion, Nucleophilic aromatic substitution (SNAr) in haloarenes is a versatile and widely used reaction in synthetic organic chemistry. The reaction mechanism involves the nucleophilic attack of a nucleophile on the electrophilic halogen atom, followed by the formation of an arenium ion and the elimination of the leaving group. The choice of nucleophile and reaction conditions can be tailored to the specific substrate and desired product, allowing for the introduction of a wide range of functional groups onto aromatic rings. SNAr in haloarenes has numerous applications in pharmaceuticals, materials science, and natural product synthesis. Overall, SNAr in haloarenes is a fundamental tool in synthetic organic chemistry and is expected to continue to play a significant role in the development of new materials and pharmaceuticals in the future.