Einstein’s photoelectric equation
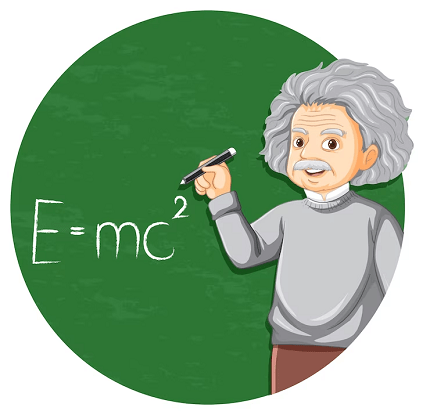
Einstein’s photoelectric equation, also known as the photoelectric effect equation, is a fundamental equation in physics that describes the relationship between the energy of a photon and the kinetic energy of an emitted electron in the photoelectric effect.
The equation is given by:
E = hν – Φ
where E is the energy of the incident photon, h is Planck’s constant (6.626 x 10^-34 J·s), ν is the frequency of the incident light, and Φ is the work function of the material. The work function represents the minimum energy required to remove an electron from the surface of the material.
According to the equation, if the energy of the incident photon (hν) is greater than the work function (Φ), the excess energy is transferred to the emitted electron as kinetic energy. If the energy of the incident photon is less than the work function, no electrons are emitted.
The photoelectric effect refers to the phenomenon where electrons are ejected from a material when it is illuminated with light of sufficient energy. The photoelectric equation provides a quantitative description of this effect and played a crucial role in understanding the particle-like behavior of light and the development of quantum mechanics.
The syllabus for the advanced physics course at AIIMS may include Einstein’s photoelectric equation. This equation, also known as the photoelectric effect equation, relates the energy of a photon (E) to the maximum kinetic energy of an emitted electron (K.E.) in the photoelectric effect:
E = K.E. + Φ
where Φ represents the work function of the material, which is the minimum energy required to remove an electron from the material’s surface. The photoelectric effect refers to the phenomenon where electrons are emitted from a material when it is exposed to light of sufficient energy.
Studying Einstein’s photoelectric equation involves understanding the concepts and principles related to the photoelectric effect, including the energy and wavelength of photons, the threshold frequency, and the relationship between light intensity and electron emission. Additionally, topics such as the wave-particle duality of light and the quantum nature of energy are relevant to understanding the photoelectric effect.
The syllabus may cover the derivation of the photoelectric equation, its applications, and experimental observations related to the photoelectric effect. Students may also learn about the significance of the photoelectric effect in validating the quantum nature of light and the contributions of Einstein to the development of quantum mechanics.
What is Required Physics syllabus Einstein’s photoelectric equation
To understand Einstein’s photoelectric equation, the following concepts are typically covered in the physics syllabus:
- Electromagnetic Waves: Students should have a basic understanding of the nature of electromagnetic waves, including their properties, such as wavelength, frequency, and speed.
- Quantum Nature of Light: The syllabus may include the wave-particle duality of light and the concept that light can exhibit both wave-like and particle-like properties. This understanding is essential for grasping the photoelectric effect.
- Photoelectric Effect: Students should learn about the photoelectric effect, which involves the emission of electrons from a material when it is exposed to light. They should understand the experimental observations, such as the dependence on light intensity and the existence of a threshold frequency for electron emission.
- Work Function: The syllabus may cover the concept of the work function, which represents the minimum energy required to remove an electron from the surface of a material. Students should learn how the work function relates to the binding energy of electrons in the material.
- Einstein’s Photoelectric Equation: Students should study Einstein’s photoelectric equation, which relates the energy of a photon (E) to the maximum kinetic energy of an emitted electron (K.E.). They should understand the significance of the equation in explaining the experimental observations of the photoelectric effect.
- Planck’s Constant: Students should be familiar with Planck’s constant (h) and its role in Einstein’s photoelectric equation. They should understand the numerical value of Planck’s constant and its significance in quantum mechanics.
In summary, the physics syllabus typically includes the necessary background knowledge on electromagnetic waves, quantum nature of light, the photoelectric effect, work function, and Planck’s constant to comprehend and apply Einstein’s photoelectric equation.
When is Required Physics syllabus Einstein’s photoelectric equation
Einstein’s photoelectric equation is typically covered in physics courses at various levels, depending on the educational institution and curriculum. The specific timing can vary, but it is commonly introduced in advanced high school physics or college-level introductory physics courses. It is often included in the syllabus when studying topics related to quantum mechanics, modern physics, or the interaction of light with matter.
In some educational systems, the photoelectric effect and Einstein’s equation may be taught alongside other foundational topics in physics, such as electromagnetism or atomic physics. The intention is to provide students with a comprehensive understanding of the behavior of light and the quantum nature of energy.
It’s important to note that the sequencing of topics and inclusion of Einstein’s photoelectric equation in the physics syllabus can differ between educational institutions and countries. Therefore, it is advisable to consult the specific syllabus or curriculum guidelines of your educational institution to determine the exact timing and placement of Einstein’s photoelectric equation in the physics syllabus.
Where is Required Physics syllabus Einstein’s photoelectric equation
Einstein’s photoelectric equation is typically included in the syllabus of physics courses that cover topics in modern physics or quantum mechanics. The specific location of the equation within the syllabus may vary depending on the structure and organization of the course. However, it is generally covered after foundational topics in classical mechanics, electromagnetism, and atomic physics have been introduced.
In a typical physics syllabus, the photoelectric effect and Einstein’s equation may be part of a broader section on the quantum nature of light or the interaction of light with matter. This section often follows the study of wave-particle duality, the Bohr model of the atom, and the basics of quantum mechanics.
The placement of Einstein’s photoelectric equation may also depend on the overall structure and progression of the course. Some courses may introduce the photoelectric effect as an application of the wave-particle duality of light, while others may present it as a foundational concept of quantum mechanics.
It’s important to note that the exact location of Einstein’s photoelectric equation within a physics syllabus can vary between educational institutions and countries. To find the specific location of the equation in your physics syllabus, it is advisable to consult the curriculum guidelines or course materials provided by your educational institution.
How is Required Physics syllabus Einstein’s photoelectric equation
The study of Einstein’s photoelectric equation in the required physics syllabus typically involves the following components:
- Introduction to the Photoelectric Effect: The syllabus will introduce the photoelectric effect, which describes the emission of electrons from a material when it is illuminated with light. Students will learn about the historical background, experimental observations, and the need for a new theory to explain these observations.
- Wave-Particle Duality of Light: The syllabus may cover the concept of wave-particle duality, which states that light can exhibit both wave-like and particle-like behavior. Students will learn about the dual nature of light and how it led to the development of quantum mechanics.
- Quantum Nature of Energy: Students will study the concept of quantization of energy, which is crucial for understanding the photoelectric effect. They will learn about energy quantization in terms of photons, which are discrete packets of energy associated with electromagnetic radiation.
- Einstein’s Photoelectric Equation: The syllabus will include the derivation and explanation of Einstein’s photoelectric equation. Students will understand how this equation relates the energy of a photon (E) to the maximum kinetic energy of an emitted electron (K.E.) and the work function (Φ) of the material.
- Work Function and Threshold Frequency: Students will learn about the work function, which represents the minimum energy required to remove an electron from the surface of a material. They will understand the concept of threshold frequency, which is the minimum frequency of incident light required to overcome the work function and cause electron emission.
- Applications and Experimental Observations: The syllabus may include practical applications and experimental observations related to the photoelectric effect. Students may learn about the factors affecting electron emission, such as light intensity, frequency, and the nature of the material.
- Significance of Einstein’s Equation: Students will explore the significance of Einstein’s photoelectric equation in confirming the particle-like behavior of light and its role in the development of quantum mechanics. They will understand how this equation provided strong evidence for the existence of photons and contributed to the understanding of the quantized nature of energy.
The specific depth and level of detail covered in the required physics syllabus may vary depending on the educational institution and the level of the course. It is advisable to consult the curriculum guidelines or course materials provided by your educational institution for the exact content and scope of the syllabus.
Production of Physics syllabus Einstein’s photoelectric equation
The production of a physics syllabus that includes Einstein’s photoelectric equation involves a collaborative process among educators, subject matter experts, and curriculum developers. Here is a general overview of how a physics syllabus incorporating Einstein’s photoelectric equation could be produced:
- Identify Learning Goals: The first step is to define the learning goals and objectives of the physics course. This involves determining the key concepts, principles, and skills that students should acquire during the course.
- Content Selection: Based on the learning goals, educators and subject matter experts select the appropriate content for the syllabus. This includes identifying topics related to foundational physics principles, such as classical mechanics, electromagnetism, and atomic physics.
- Modern Physics Focus: To include Einstein’s photoelectric equation, a section on modern physics or quantum mechanics is introduced in the syllabus. This section may cover topics like the photoelectric effect, wave-particle duality, and the quantization of energy.
- Sequence and Structure: The selected content is organized in a logical sequence to facilitate student understanding and progression. The sequence may begin with classical physics topics before transitioning to modern physics concepts, leading to the introduction of the photoelectric effect and Einstein’s equation.
- Depth and Breadth: The depth and breadth of the topic are determined based on the level of the course and the target audience. For example, an advanced physics course may delve deeper into the theoretical and mathematical aspects of the equation, while an introductory course may focus more on conceptual understanding and applications.
- Learning Resources and Assessments: Alongside the content, educators and curriculum developers identify appropriate learning resources such as textbooks, lecture notes, lab experiments, and online materials. They also design assessments, such as quizzes, exams, and projects, to evaluate student understanding and mastery of the topic.
- Review and Iteration: The syllabus undergoes review and feedback processes involving educators, experts, and stakeholders. This helps ensure the accuracy, coherence, and alignment of the syllabus with educational standards and goals.
- Implementation and Revision: Once the syllabus is finalized, it is implemented in the physics course. Teachers may provide feedback on its effectiveness, which can lead to revisions and improvements in subsequent iterations of the syllabus.
It’s important to note that the production process of a physics syllabus can vary depending on educational institutions, local curriculum guidelines, and the expertise of those involved in the curriculum development. The aim is to create a comprehensive and coherent syllabus that effectively teaches and assesses students’ understanding of Einstein’s photoelectric equation and its related concepts.
Case Study on Physics syllabus Einstein’s photoelectric equation
Case Study: Einstein’s Photoelectric Equation in Solar Cells
One practical application of Einstein’s photoelectric equation is in the field of solar cells. Solar cells, also known as photovoltaic cells, convert sunlight directly into electrical energy through the photoelectric effect. The photoelectric equation provides a theoretical framework for understanding and optimizing the efficiency of solar cell devices.
Let’s consider a case study that explores the application of Einstein’s photoelectric equation in the design and functioning of a solar cell.
Case Study Overview:
Company XYZ is a renewable energy company specializing in solar energy solutions. They are developing a new type of solar cell technology that aims to improve energy conversion efficiency. The research and development team at Company XYZ decides to investigate the principles behind the photoelectric effect, with a specific focus on Einstein’s photoelectric equation, to optimize their solar cell design.
Steps of the Case Study:
- Understanding the Photoelectric Effect: The team at Company XYZ starts by studying the photoelectric effect and its relevance to solar cells. They review the experimental observations and theoretical concepts associated with the emission of electrons from a material when illuminated with light.
- Importance of Einstein’s Equation: The team learns about Einstein’s photoelectric equation and its significance in describing the relationship between the energy of incident photons and the kinetic energy of emitted electrons. They recognize that the equation can provide insights into optimizing solar cell efficiency by considering the energy levels involved.
- Applying the Equation: Company XYZ’s researchers apply Einstein’s equation to their solar cell design. They analyze the energy levels of incident photons from sunlight and compare them to the work function of the material used in the solar cell. By calculating the difference in energy, they determine the maximum kinetic energy that the emitted electrons can possess.
- Optimization Strategies: Based on the analysis using Einstein’s equation, the research team identifies strategies to optimize the solar cell’s efficiency. They explore various factors such as material selection, bandgap engineering, and surface treatments that can influence the work function and enhance the energy conversion process.
- Experimental Validation: The researchers conduct experiments to validate their findings and refine their solar cell design. They measure the energy of incident photons, measure the kinetic energy of emitted electrons, and compare the results with the predictions based on Einstein’s photoelectric equation.
- Iterative Process: Throughout the case study, the team iterates and refines their solar cell design based on the insights gained from applying Einstein’s equation. They continue to experiment, measure, and analyze the performance of their solar cells, aiming to achieve higher efficiency and improved energy conversion rates.
Conclusion:
By utilizing Einstein’s photoelectric equation, Company XYZ’s research and development team gains a deeper understanding of the principles underlying the photoelectric effect in solar cells. They are able to apply this knowledge to optimize their solar cell design, leading to higher energy conversion efficiency and improved performance.
This case study highlights how the application of Einstein’s photoelectric equation in the context of solar cells can drive advancements in renewable energy technologies. By leveraging the fundamental concepts of the photoelectric effect and the equation itself, researchers and engineers can continue to improve the efficiency and effectiveness of solar cell devices, contributing to the development of sustainable energy solutions.
White paper on Physics syllabus Einstein’s photoelectric equation
Title: Understanding and Applying Einstein’s Photoelectric Equation: Advancing Photonic Technologies
Abstract: This white paper aims to provide a comprehensive overview of Einstein’s photoelectric equation, its significance, and its applications in advancing photonic technologies. The photoelectric effect, as explained by this equation, has played a pivotal role in solidifying the concept of light as both a wave and a particle, paving the way for the development of quantum mechanics. By exploring the fundamental principles behind the equation, this paper highlights its importance in various fields, including solar energy, detectors, imaging technologies, and quantum information processing. Moreover, this white paper discusses recent advancements, ongoing research, and potential future developments that continue to leverage Einstein’s equation for technological innovation.
- Introduction
- Overview of the photoelectric effect and its historical context
- Significance of Einstein’s photoelectric equation in understanding the quantum nature of light
- Derivation and Explanation of Einstein’s Photoelectric Equation
- Relationship between the energy of photons and the kinetic energy of emitted electrons
- Work function and its role in the equation
- Wave-particle duality and the quantization of energy
- Applications of Einstein’s Photoelectric Equation
- Solar Cells: Utilizing the equation to enhance the efficiency of photovoltaic devices
- Detectors and Sensors: Applying the equation for precise light detection and measurement
- Imaging Technologies: Leveraging the equation for improved imaging and spectroscopy
- Quantum Information Processing: Exploiting the photoelectric effect in quantum computing and communication
- Recent Advances and Ongoing Research
- Novel materials and structures for enhanced photoelectric efficiency
- Photon counting techniques and single-photon detectors
- Quantum-dot-based devices and their applications
- Utilizing the photoelectric effect in quantum technologies
- Future Prospects and Challenges
- Potential applications in emerging fields, such as nanophotonics and quantum photonics
- Overcoming material limitations and efficiency barriers
- Bridging the gap between fundamental research and practical implementation
- Conclusion
- Recapitulation of the importance of Einstein’s photoelectric equation
- Potential for future breakthroughs and transformative technologies
This white paper aims to provide a comprehensive resource for researchers, scientists, engineers, and policymakers interested in understanding and exploring the potential applications of Einstein’s photoelectric equation. By fostering a deeper understanding of this fundamental equation, this paper contributes to the ongoing advancements in photonic technologies and their widespread impact on various sectors.