Matter waves
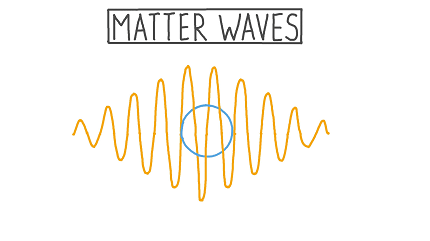
Matter waves, also known as de Broglie waves, refer to the wave-like behavior exhibited by particles at the microscopic level, such as electrons, protons, and even larger particles like atoms and molecules. The concept of matter waves is based on Louis de Broglie’s hypothesis that particles can possess both particle and wave characteristics.
According to de Broglie’s hypothesis, every particle has an associated wavelength, known as the de Broglie wavelength, which is inversely proportional to its momentum. The de Broglie wavelength can be calculated using the equation λ = h/p, where λ is the wavelength, h is Planck’s constant, and p is the momentum of the particle.
The wave-like nature of particles is evident in phenomena such as diffraction and interference. For example, in the famous Davisson-Germer experiment, electrons were diffracted by a crystal lattice, demonstrating that electrons exhibit wave-like properties. This experiment provided experimental evidence for the wave-particle duality principle.
Matter wave interference occurs when two or more matter waves overlap and interact. Similar to the interference of light waves, matter waves can exhibit constructive interference (resulting in regions of enhanced intensity) or destructive interference (resulting in regions of reduced or zero intensity). The principle of superposition applies to matter waves, allowing the combination of multiple wave functions.
The understanding of matter waves has led to various applications in science and technology. For instance, electron microscopy utilizes the wave nature of electrons to achieve high-resolution imaging of microscopic structures. Matter waves also play a role in understanding the behavior of particles in quantum mechanics and are fundamental to the study of quantum mechanics and its applications.
In summary, matter waves describe the wave-like behavior exhibited by particles and are a significant aspect of quantum mechanics, providing insights into the dual nature of matter.
The Physics syllabus of the advanced course at AIIMS includes the topic of matter waves. Matter waves refer to the wave-like properties exhibited by particles, such as electrons and other microscopic entities. The syllabus covers the following aspects of matter waves:
- de Broglie wavelength: The concept of matter waves was introduced by Louis de Broglie, who proposed that particles can exhibit wave-like behavior. The syllabus covers the de Broglie wavelength equation, which relates the wavelength of a particle to its momentum.
- Wave-particle duality: The syllabus explores the wave-particle duality principle, which states that particles can exhibit both particle-like and wave-like properties. Matter waves provide an understanding of this duality, where particles can be described as both particles and waves simultaneously.
- Davisson-Germer experiment: The syllabus includes the Davisson-Germer experiment, which demonstrated the diffraction of electrons, providing experimental evidence for the wave nature of particles. Students will learn about the setup and the results obtained from this famous experiment.
- Matter wave interference: The syllabus covers the phenomenon of interference exhibited by matter waves. Students will learn about the conditions required for interference, including the concept of phase difference and the principle of superposition.
- Applications of matter waves: The syllabus may also include applications of matter waves, such as electron microscopy, where the wave nature of electrons is utilized to image microscopic objects with high resolution.
These are the key topics related to matter waves that you can expect to study in the Physics syllabus of the advanced course at AIIMS.
What is Required Physics syllabus Matter waves
The required Physics syllabus for matter waves typically includes the following key topics:
- de Broglie wavelength: The concept of de Broglie wavelength and its relationship with the momentum of particles.
- Wave-particle duality: Understanding the wave-particle duality principle, which states that particles can exhibit both particle-like and wave-like properties.
- Davisson-Germer experiment: Studying the famous Davisson-Germer experiment that demonstrated the diffraction of electrons, providing evidence for the wave nature of particles.
- Diffraction and interference: Exploring the phenomenon of diffraction and interference of matter waves, including the conditions for interference, the principle of superposition, and the concept of phase difference.
- Applications of matter waves: Understanding the applications of matter waves, such as electron microscopy, which utilizes the wave nature of electrons to image microscopic objects with high resolution.
It’s important to note that the specific syllabus may vary depending on the educational institution and the level of the course. It’s recommended to refer to the official syllabus or consult the course instructor for the most accurate and detailed information regarding the required Physics syllabus on matter waves.
When is Required Physics syllabus Matter waves
The study of matter waves is typically included in the Physics syllabus at various educational levels. The specific timing of when matter waves are covered in the syllabus can vary depending on the curriculum and educational institution. However, matter waves are commonly taught in the context of quantum mechanics or modern physics courses.
At the high school level, matter waves may be introduced in advanced Physics courses or as part of an optional module in the curriculum. It is often covered after the foundational topics of classical mechanics, electromagnetism, and optics.
In undergraduate physics programs, matter waves are typically covered in courses specifically dedicated to quantum mechanics or modern physics. These courses are usually taken in the second or third year of undergraduate studies, following introductory physics and mathematics courses.
In postgraduate or advanced-level physics programs, matter waves are usually revisited and explored in greater detail as part of advanced quantum mechanics or specialized courses in quantum field theory or atomic and molecular physics.
It’s important to check the specific syllabus or course outline of your educational institution to determine the exact timing and inclusion of matter waves in the Physics curriculum.
Where is Required Physics syllabus Matter waves
The required Physics syllabus covering matter waves can be found in various educational settings, including schools, colleges, and universities. The specific location within the curriculum can vary depending on the educational system and level of study. Here are some common places where matter waves may be included:
- High school physics: Matter waves are often introduced in advanced or honors physics courses at the high school level. They may be covered as part of a unit on modern physics or quantum mechanics.
- Undergraduate physics programs: Matter waves are typically included in undergraduate physics programs as part of courses dedicated to quantum mechanics or modern physics. These courses are often taken in the second or third year of undergraduate studies.
- Graduate physics programs: Matter waves may also be revisited and studied in greater depth in graduate-level physics programs. Advanced courses in quantum mechanics, atomic and molecular physics, or quantum field theory may cover matter waves as part of their syllabus.
- Entrance exams: Some entrance exams for higher education in physics, such as those for graduate school admissions, may include questions on matter waves. In such cases, the syllabus may be specified in the exam notification or guidelines.
To find the exact location of matter waves in the physics syllabus, it is best to consult the official curriculum or syllabus document provided by the educational institution or refer to the course outlines and descriptions for relevant physics courses.
How is Required Physics syllabus Matter waves
The required Physics syllabus on matter waves typically involves studying the fundamental concepts and properties of matter waves. Here is a general outline of how matter waves are covered in the syllabus:
- Introduction to matter waves: The syllabus begins with an introduction to the concept of matter waves and the wave-particle duality. Students learn about the historical background and the contributions of scientists like Louis de Broglie.
- De Broglie wavelength: The syllabus covers the de Broglie wavelength equation, which relates the wavelength of a particle to its momentum. Students learn how to calculate the de Broglie wavelength for different particles, such as electrons and protons.
- Wave-like properties: The syllabus explores the wave-like properties exhibited by matter waves. This includes topics like diffraction, interference, and the principle of superposition. Students learn about the conditions required for diffraction and interference to occur, and how the wave nature of particles can be observed in various experiments.
- Experimental evidence: The syllabus includes the study of experimental evidence supporting the wave nature of particles. This may involve discussing experiments like the Davisson-Germer experiment, where electrons were diffracted by a crystal lattice, providing confirmation of the wave-like behavior of particles.
- Applications of matter waves: The syllabus may also cover practical applications of matter waves. This could include topics like electron microscopy, where the wave nature of electrons is utilized for imaging microscopic structures with high resolution.
The specific depth and detail of each topic within the syllabus can vary depending on the educational level and institution. It’s important to refer to the official curriculum or syllabus provided by the educational institution to get a comprehensive understanding of how matter waves are covered in the required Physics syllabus.
Structures of Physics syllabus Matter waves
The structure of the Physics syllabus on matter waves may vary depending on the educational level and institution. However, here is a typical structure that covers the key concepts and topics related to matter waves:
- Introduction to matter waves:
- Historical development and significance of matter waves
- Concept of wave-particle duality and its implications
- Wave nature of particles:
- de Broglie hypothesis and de Broglie wavelength equation
- Relationship between wavelength, momentum, and energy of particles
- Calculations and examples of de Broglie wavelengths for different particles
- Diffraction and interference of matter waves:
- Basics of diffraction and interference phenomena
- Conditions for diffraction and interference in matter waves
- Diffraction experiments with electrons, neutrons, or other particles
- Interference patterns and the principle of superposition for matter waves
- Experimental evidence and phenomena:
- Davisson-Germer experiment and the discovery of electron diffraction
- Other experimental evidence supporting the wave nature of particles
- Wave-like behavior observed in various experiments (e.g., single and double slit experiments)
- Applications and implications of matter waves:
- Electron microscopy and its principles
- Quantum mechanical interpretation of matter waves
- Quantum mechanics and the wave function description of particles
It’s important to note that the actual syllabus structure can vary. The depth of coverage, inclusion of mathematical derivations, and additional related topics may depend on the educational level (high school, undergraduate, or graduate) and the specific curriculum of the institution.
To have a precise understanding of the Physics syllabus on matter waves, it’s recommended to consult the official curriculum or syllabus documents provided by the educational institution or refer to the course outlines and descriptions specific to the course you are enrolled in.
Case Study on Physics syllabus Matter waves
Case Study: Electron Diffraction and Matter Waves
Introduction: In the early 20th century, the wave-particle duality of matter was a subject of intense investigation. One of the key experiments that provided evidence for the wave nature of particles, specifically electrons, was the Davisson-Germer experiment. This case study explores the experiment and its implications for the understanding of matter waves.
Background: In 1927, Clinton Davisson and Lester Germer conducted an experiment at Bell Labs in the United States. They aimed to investigate the behavior of electrons when they interacted with a crystal surface. The prevailing understanding at the time was that electrons behaved solely as particles, similar to tiny billiard balls.
Experimental Setup: The experimental setup consisted of a heated tungsten filament that emitted a beam of electrons. The electrons were accelerated through a high voltage and then directed towards a nickel crystal. The crystal surface was carefully prepared and positioned in the path of the electron beam. A detector was placed at an angle to the incident beam to measure the scattered electrons.
Experimental Observations: Davisson and Germer observed unexpected and intriguing results in their experiment. They found that the electrons exhibited a diffraction pattern when they interacted with the nickel crystal. The scattered electrons formed a distinct pattern of bright and dark spots on the detector screen, similar to the patterns observed in the diffraction of light waves.
Implications and Interpretation: The diffraction pattern observed in the Davisson-Germer experiment could not be explained by considering electrons solely as particles. Instead, it indicated that electrons possessed wave-like characteristics, thus supporting the wave-particle duality concept proposed by Louis de Broglie.
The experiment provided direct evidence for the existence of matter waves and the de Broglie hypothesis. It showed that electrons could behave as waves and undergo diffraction, similar to how light waves diffract when passing through narrow slits or around obstacles.
The diffraction pattern observed in the Davisson-Germer experiment confirmed that the wavelength of electrons could be determined using the de Broglie equation, which relates the wavelength (λ) to the momentum (p) of particles: λ = h/p, where h is Planck’s constant.
Significance and Further Developments: The Davisson-Germer experiment was a groundbreaking achievement, and both Clinton Davisson and George Paget Thomson, who independently performed similar experiments, were awarded the Nobel Prize in Physics in 1937 for their work on electron diffraction.
This experiment had profound implications for the development of quantum mechanics and the understanding of the dual nature of particles. It provided strong experimental evidence supporting the wave-particle duality principle and paved the way for further exploration of matter waves in various fields, including quantum mechanics, solid-state physics, and electron microscopy.
Conclusion: The Davisson-Germer experiment stands as a landmark case study in the exploration of matter waves. By demonstrating the diffraction of electrons and providing experimental evidence for their wave-like behavior, the experiment solidified the wave-particle duality concept and opened up new avenues of research in the field of quantum physics.
White paper on Physics syllabus Matter waves
Title: Matter Waves: Exploring the Wave-Particle Duality
Abstract: This white paper delves into the fascinating concept of matter waves and the wave-particle duality in the realm of physics. We explore the historical background, fundamental principles, experimental evidence, and implications of matter waves. Understanding matter waves is crucial for grasping the true nature of particles and their behavior, which has revolutionized our understanding of quantum mechanics and opened up new avenues for technological advancements.
- Introduction:
- Brief overview of the wave-particle duality concept
- Significance of matter waves in quantum mechanics
- Historical Development:
- Contributions of Louis de Broglie and his hypothesis
- Early experiments and observations supporting matter waves
- De Broglie Wavelength and Particle Properties:
- De Broglie equation and its significance
- Relationship between wavelength, momentum, and energy
- Calculation of de Broglie wavelengths for different particles
- Diffraction and Interference of Matter Waves:
- Explanation of diffraction and interference phenomena
- Conditions for diffraction and interference in matter waves
- Notable experiments demonstrating diffraction and interference effects
- Experimental Evidence and Observations:
- Davisson-Germer experiment and the discovery of electron diffraction
- Other experiments confirming the wave-like behavior of particles
- Quantum mechanical interpretations and predictions based on matter waves
- Applications and Technological Implications:
- Electron microscopy and its high-resolution imaging capabilities
- Matter wave interferometry and its applications in precision measurements
- Matter wave-based technologies and future prospects
- Quantum Mechanics and Matter Waves:
- Incorporation of matter waves in the Schrödinger equation
- Wave function description and probability interpretation
- Quantum mechanical implications and predictions based on matter waves
- Future Directions and Open Questions:
- Ongoing research and advancements in matter wave studies
- Unresolved questions and challenges in understanding matter waves
- Potential future applications and technological breakthroughs
- Conclusion:
- Recap of the importance of matter waves in modern physics
- Key takeaways and implications for the wave-particle duality concept
- Potential for further exploration and advancements in matter wave research
This white paper aims to provide a comprehensive overview of matter waves, their experimental evidence, and their significance in our understanding of particles and quantum mechanics. By exploring the wave-particle duality, we gain deeper insights into the fundamental nature of matter and lay the groundwork for future scientific discoveries and technological advancements.