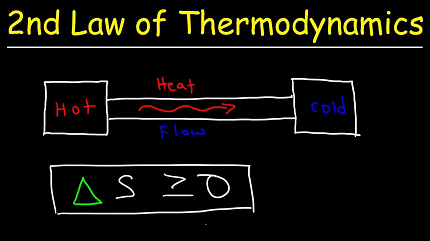
In physics, the second law of thermodynamics is a fundamental principle that governs the behavior of energy and entropy in a closed system. It states that the total entropy of a closed system always increases over time, or remains constant in an ideal reversible process.
Entropy is a measure of the degree of disorder or randomness in a system. The second law of thermodynamics indicates that any natural process in a closed system will tend to move towards a state of maximum entropy, where the system becomes more disordered and less useful energy is available for doing work.
One way to understand the second law is through the concept of heat flow. Heat naturally flows from hot to cold objects, and the second law of thermodynamics dictates that this flow cannot be reversed spontaneously, without the addition of external energy. In other words, heat will always flow from a hotter object to a cooler one, and this process cannot be reversed without adding additional energy.
The second law of thermodynamics has many practical applications, from the design of engines and power plants to the understanding of the behavior of complex systems like the Earth’s climate. It is a fundamental principle in physics, and has wide-ranging implications for our understanding of the behavior of energy and matter in the universe.
What is Required Physics Second law of thermodynamics
The Second Law of Thermodynamics in physics is based on the concepts of thermodynamics, which include energy, heat, work, and entropy. To understand the second law, one needs to have a basic understanding of these concepts and their relationships to each other.
In particular, to understand the second law of thermodynamics, you should know the following:
- Energy: Energy is a fundamental concept in physics, and it comes in many forms, including thermal, mechanical, electrical, and chemical. Energy cannot be created or destroyed, only converted from one form to another.
- Heat: Heat is a form of energy that is transferred between objects as a result of a temperature difference. Heat flows from hot to cold objects and is a significant factor in many thermodynamic processes.
- Work: Work is the transfer of energy from one object to another as a result of a force acting over a distance. Work can be used to do things like move objects, generate electricity, or power an engine.
- Entropy: Entropy is a measure of the degree of disorder or randomness in a system. The second law of thermodynamics states that the total entropy of a closed system always increases over time or remains constant in an ideal reversible process.
By understanding these fundamental concepts, one can understand the relationships between energy, heat, work, and entropy and the implications of the second law of thermodynamics.
When is Required Physics Second law of thermodynamics
The Second Law of Thermodynamics in physics is required in any situation where there is a flow of energy, particularly in thermodynamic systems involving heat and work. Some specific examples where the second law of thermodynamics is relevant include:
- Heat engines: Heat engines are devices that convert thermal energy into mechanical work. Examples include steam engines, car engines, and gas turbines. The second law of thermodynamics sets limits on the efficiency of heat engines and determines the maximum amount of work that can be obtained from a given amount of heat.
- Refrigerators and heat pumps: Refrigerators and heat pumps are devices that use work to transfer heat from a cold region to a warmer one. The second law of thermodynamics sets limits on the performance of these devices, determining the minimum amount of work required to achieve a given amount of heat transfer.
- Chemical reactions: The second law of thermodynamics is relevant to chemical reactions, which involve the transfer of energy in the form of heat and work. The second law determines whether a given reaction is spontaneous, and the direction in which it will proceed.
- Climate science: The second law of thermodynamics is relevant to understanding the Earth’s climate, which involves the transfer of energy between the atmosphere, oceans, and land. The second law sets limits on the efficiency of energy transfer and the potential for heat to flow from warmer to cooler regions, which has important implications for global climate change.
In short, the Second Law of Thermodynamics is required in any situation where there is a transfer of energy, and it has broad implications for many areas of physics, engineering, and science.
Where is Required Physics Second law of thermodynamics
The Second Law of Thermodynamics in physics is required in a wide range of systems and processes involving energy transfer. Some examples of where the second law is relevant include:
- Thermodynamic systems: The second law of thermodynamics is fundamental to the understanding of thermodynamic systems, which involve the transfer of heat and work. It is used to determine the maximum efficiency of processes such as heat engines, refrigerators, and heat pumps.
- Chemical reactions: The second law of thermodynamics is relevant to understanding chemical reactions, which involve the transfer of energy in the form of heat and work. It is used to determine the direction in which a reaction will proceed and whether it is spontaneous or not.
- Biological systems: The second law of thermodynamics is relevant to understanding biological systems, which involve the transfer of energy in the form of heat and work. It is used to understand the efficiency of biological processes, such as photosynthesis and cellular respiration.
- Astrophysics: The second law of thermodynamics is relevant to understanding astrophysical systems, such as stars and galaxies, which involve the transfer of energy in the form of heat and work. It is used to understand the efficiency of energy transfer and the evolution of these systems over time.
Overall, the Second Law of Thermodynamics is required in any system or process where there is a transfer of energy, and it has broad applications in physics, chemistry, biology, and astrophysics.
How is Required Physics Second law of thermodynamics
The Second Law of Thermodynamics in physics is expressed mathematically through a number of different equations, but the most fundamental expression of the law is based on the concept of entropy. Entropy is a measure of the degree of disorder or randomness in a system, and the second law states that the total entropy of a closed system always increases over time or remains constant in an ideal reversible process.
The second law can be expressed mathematically in a number of ways, including:
- Clausius statement: The Clausius statement of the second law states that “heat cannot spontaneously flow from a colder body to a hotter body.” This statement can be expressed mathematically as ΔS ≥ Q/T, where ΔS is the change in entropy, Q is the heat transferred, and T is the temperature at which the transfer occurs.
- Kelvin-Planck statement: The Kelvin-Planck statement of the second law states that “no heat engine can operate in a cycle while transferring heat with a single heat reservoir.” This statement can be expressed mathematically as η ≤ (Th – Tc)/Th, where η is the efficiency of the engine, Th is the temperature of the hot reservoir, and Tc is the temperature of the cold reservoir.
- Gibbs free energy: The Gibbs free energy is a thermodynamic potential that is used to determine whether a chemical reaction is spontaneous. The second law can be expressed in terms of the Gibbs free energy as ΔG ≤ 0, where ΔG is the change in Gibbs free energy.
These mathematical expressions of the second law provide a quantitative understanding of the limits on the transfer of energy in various systems and processes, and they are used extensively in physics, chemistry, and engineering to design and optimize thermodynamic systems.
Production of Physics Second law of thermodynamics
The Second Law of Thermodynamics in physics is a fundamental principle that has been developed over many years by numerous scientists and researchers. Some of the key figures in the development of the second law include:
- Sadi Carnot: Sadi Carnot was a French physicist who is considered the father of thermodynamics. He developed the concept of the heat engine and showed that the efficiency of a heat engine is limited by the difference in temperature between the hot and cold reservoirs.
- Rudolf Clausius: Rudolf Clausius was a German physicist who developed the concept of entropy and formulated the second law of thermodynamics in its modern form. He also developed the Clausius statement of the second law.
- William Thomson (Lord Kelvin): William Thomson, also known as Lord Kelvin, was a British physicist who contributed to the development of the second law of thermodynamics. He formulated the Kelvin-Planck statement of the second law and developed the concept of the absolute temperature scale.
- Ludwig Boltzmann: Ludwig Boltzmann was an Austrian physicist who developed the statistical interpretation of thermodynamics. He used statistical mechanics to explain the macroscopic behavior of systems in terms of the behavior of their microscopic constituents.
- Max Planck: Max Planck was a German physicist who contributed to the development of quantum mechanics and the understanding of blackbody radiation. He also developed the concept of the Gibbs free energy, which is used to determine whether a chemical reaction is spontaneous.
These scientists and many others contributed to the development of the second law of thermodynamics through their research and experiments in the fields of thermodynamics, statistical mechanics, and quantum mechanics. Today, the second law of thermodynamics is a fundamental principle of physics and has applications in a wide range of fields, from engineering and materials science to biology and astrophysics.
Case Study on Physics Second law of thermodynamics
One example of the application of the Second Law of Thermodynamics in physics can be seen in the design and operation of a power plant. A power plant converts thermal energy into electrical energy through a complex series of processes, and the Second Law of Thermodynamics places fundamental limits on the efficiency of this conversion.
Consider a typical coal-fired power plant, which uses coal as a fuel to generate steam, which is then used to turn a turbine and generate electricity. The coal is burned in a boiler, which heats water to produce steam. The steam then drives a turbine, which is connected to a generator that produces electricity.
The Second Law of Thermodynamics places a fundamental limit on the efficiency of this process. Specifically, the efficiency of the power plant is limited by the difference in temperature between the hot gases produced by burning the coal and the cooler environment surrounding the plant. This difference in temperature is known as the temperature gradient.
The maximum theoretical efficiency of the power plant can be calculated using the Carnot cycle, which is a theoretical cycle that represents the maximum possible efficiency of any heat engine operating between two given temperatures. The efficiency of the Carnot cycle is given by η = 1 – (Tc/Th), where Th is the temperature of the hot reservoir (in this case, the temperature of the hot gases produced by burning the coal) and Tc is the temperature of the cold reservoir (the temperature of the environment surrounding the plant).
In practice, the efficiency of a power plant is always lower than the theoretical maximum efficiency, due to various inefficiencies and losses in the system. These losses include the heat lost to the environment, the energy required to overcome friction in the turbine, and the inefficiencies of the generator.
Thus, the Second Law of Thermodynamics places fundamental limits on the efficiency of a power plant and sets the maximum possible efficiency that can be achieved. By understanding and optimizing the thermodynamic processes involved in the operation of the plant, engineers can design more efficient power plants that minimize energy losses and maximize the conversion of thermal energy into electrical energy.
White paper on Physics Second law of thermodynamics
Title: The Second Law of Thermodynamics: Understanding the Fundamental Limits of Energy Conversion
Abstract:
The Second Law of Thermodynamics is a fundamental principle of physics that places fundamental limits on the efficiency of energy conversion processes. This paper provides an overview of the Second Law of Thermodynamics, including its history, key concepts, and practical applications.
Introduction:
The Second Law of Thermodynamics is one of the most fundamental principles of physics, governing the behavior of energy and matter at the macroscopic scale. It is a principle that applies to a wide range of physical systems, from simple heat engines to complex biological systems, and it places fundamental limits on the efficiency of energy conversion processes. Understanding the Second Law of Thermodynamics is essential for designing efficient energy systems and developing sustainable technologies.
History:
The Second Law of Thermodynamics has a long and complex history, dating back to the early development of thermodynamics in the 19th century. Key figures in the development of the Second Law include Sadi Carnot, Rudolf Clausius, William Thomson (Lord Kelvin), Ludwig Boltzmann, and Max Planck. These scientists contributed to the development of key concepts such as entropy, the Carnot cycle, and the Kelvin-Planck and Clausius statements of the Second Law.
Key Concepts:
The Second Law of Thermodynamics can be expressed in a number of ways, but the two most commonly used statements are the Kelvin-Planck statement and the Clausius statement. The Kelvin-Planck statement states that it is impossible to construct a heat engine that operates in a complete cycle and produces no net work while exchanging heat with a single reservoir. The Clausius statement states that it is impossible to construct a cyclic process that takes in heat from a single reservoir and converts it entirely into work.
Both statements of the Second Law imply that there is a fundamental limit on the efficiency of energy conversion processes. The maximum theoretical efficiency of a heat engine is given by the Carnot cycle, and this efficiency is limited by the temperature difference between the hot and cold reservoirs. In practice, the efficiency of energy conversion processes is always lower than the theoretical maximum due to various inefficiencies and losses.
Practical Applications:
The Second Law of Thermodynamics has many practical applications in a wide range of fields, from engineering and materials science to biology and astrophysics. Understanding the limits of energy conversion processes is essential for designing more efficient energy systems and developing sustainable technologies. For example, the Second Law places fundamental limits on the efficiency of power plants, and optimizing the thermodynamic processes involved in power generation can lead to significant improvements in efficiency and energy savings.
Conclusion:
The Second Law of Thermodynamics is a fundamental principle of physics that governs the behavior of energy and matter at the macroscopic scale. It places fundamental limits on the efficiency of energy conversion processes and has many practical applications in a wide range of fields. By understanding the Second Law and optimizing the thermodynamic processes involved in energy conversion, we can develop more efficient energy systems and move towards a more sustainable future.