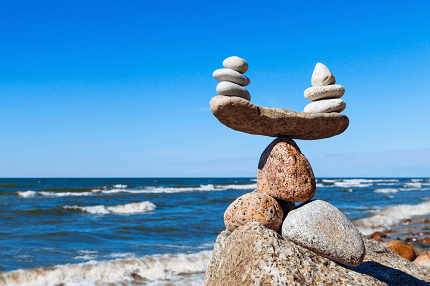
Stability refers to the ability of a system, structure, or entity to maintain its equilibrium or resist being disturbed or changed. In different fields, the concept of stability can have different meanings and applications.
In physics, stability refers to the ability of an object to maintain its position or motion in the face of external forces. For example, a ball at the bottom of a bowl is stable because it will return to its resting position if disturbed. In contrast, a ball balanced on top of a narrow pole is unstable because it will fall over with even a small disturbance.
In chemistry, stability refers to the tendency of a compound or mixture to resist decomposition or reaction under certain conditions. For example, a compound that is thermodynamically stable will not undergo spontaneous decomposition or reaction unless the conditions are changed.
In economics, stability refers to the ability of a system, such as a financial market or an economy, to maintain a steady state or avoid large fluctuations in key variables such as prices, output, or employment.
Overall, stability is a critical concept in many areas of science and engineering, and it is often desirable to design systems or structures that are as stable as possible to ensure their longevity and reliability.
What is Required Coordination Compounds Stability
The stability of coordination compounds is determined by several factors, including the nature of the central metal ion, the identity and nature of the ligands attached to the metal, and the overall geometry of the complex.
The nature of the metal ion plays a crucial role in determining the stability of coordination compounds. Generally, transition metals with partially filled d-orbitals can form stable coordination compounds, while metals with completely filled d-orbitals do not. This is because the partially filled d-orbitals can interact with the ligands and form strong coordination bonds.
The identity and nature of the ligands attached to the metal ion also play a critical role in determining the stability of coordination compounds. Ligands that are electron-rich and have lone pairs of electrons, such as amines and phosphines, tend to form more stable complexes than those that are electron-poor, such as halides and cyanides.
The overall geometry of the complex also affects the stability of coordination compounds. Octahedral complexes with a high degree of symmetry tend to be more stable than complexes with lower symmetry or distorted geometries.
In addition to these factors, the stability of coordination compounds can also be affected by environmental factors, such as pH, temperature, and the presence of other ions in solution.
Overall, a combination of these factors determines the stability of coordination compounds, and understanding these factors is essential for designing and synthesizing stable coordination compounds for use in various applications, such as catalysis, sensing, and medicine.
When is Required Coordination Compounds Stability
Coordination compounds are important in a variety of applications, including catalysis, medicine, materials science, and sensing. The stability of coordination compounds is important in all of these applications, as it determines their effectiveness and longevity.
In catalysis, for example, stable coordination compounds are necessary to ensure that the catalyst remains active over long periods of time. Catalysts based on unstable or poorly coordinated complexes may decompose or lose activity quickly, reducing their effectiveness.
In medicine, stable coordination compounds are important for ensuring the safety and efficacy of drugs. Coordination compounds can be used to target specific tissues or cells in the body, and their stability is critical for controlling the release of the drug and preventing side effects.
In materials science, stable coordination compounds can be used to create new materials with specific properties, such as magnetism or conductivity. The stability of the coordination complex determines the overall structure and properties of the material, making it important to design and synthesize stable compounds for specific applications.
In sensing, stable coordination compounds can be used as probes to detect specific molecules or ions in solution. The stability of the complex is important for ensuring that the probe remains active and selective over time.
Overall, the stability of coordination compounds is important in a variety of applications, and understanding the factors that affect stability is essential for designing and synthesizing effective and long-lasting coordination compounds.
Where is Required Coordination Compounds Stability
The stability of coordination compounds is important in various fields, including chemistry, biochemistry, materials science, medicine, and environmental science. In these fields, coordination compounds are used for a wide range of applications, including catalysis, drug design, materials synthesis, and environmental sensing.
In chemistry, coordination compounds are used to study the properties of metals and ligands, as well as to explore new reactions and mechanisms. Coordination compounds can also be used as precursors for the synthesis of new materials, such as metal-organic frameworks (MOFs) and coordination polymers.
In biochemistry, coordination compounds are used to study the role of metal ions in biological processes, as well as to design metal-based drugs and probes for imaging and sensing. Metal ions such as iron, copper, and zinc are essential for many biological processes, and coordination compounds can be used to mimic these processes and study their mechanisms.
In materials science, coordination compounds are used to create new materials with specific properties, such as porosity, magnetism, and conductivity. Coordination compounds can be used as precursors for the synthesis of metal oxides, nanoparticles, and other advanced materials.
In medicine, coordination compounds are used to design metal-based drugs for the treatment of cancer, arthritis, and other diseases. Coordination compounds can also be used as imaging probes for the diagnosis of diseases and as sensors for detecting biological molecules.
In environmental science, coordination compounds are used to detect and remove pollutants from water and air. Coordination compounds can be designed to selectively bind to specific pollutants, making them useful for environmental sensing and remediation.
Overall, the stability of coordination compounds is important in many different fields and applications, and understanding the factors that affect stability is essential for designing and synthesizing effective and long-lasting coordination compounds.
How is Required Coordination Compounds Stability
The stability of coordination compounds can be influenced by a variety of factors, including the nature of the central metal ion, the identity and nature of the ligands attached to the metal, and the overall geometry of the complex. Here are some of the ways in which each of these factors can affect the stability of coordination compounds:
- Nature of the metal ion: The stability of coordination compounds is often dependent on the size and electronic properties of the central metal ion. Metals with partially filled d-orbitals, such as the transition metals, are generally more likely to form stable coordination compounds, as they can interact strongly with the ligands. The oxidation state of the metal ion also plays a role, as higher oxidation states can lead to more stable coordination compounds due to the greater electronegativity of the metal ion.
- Identity and nature of the ligands: The nature of the ligands attached to the metal ion is critical for determining the stability of the coordination complex. Electron-rich ligands, such as amines and phosphines, tend to form more stable complexes than electron-poor ligands, such as halides and cyanides. The size and shape of the ligands also play a role, as larger or more bulky ligands can lead to steric hindrance that may destabilize the complex.
- Geometry of the complex: The overall geometry of the coordination complex is also important for determining its stability. Complexes with higher symmetry and regular geometries, such as octahedral complexes, are generally more stable than those with lower symmetry or distorted geometries. This is because regular geometries can minimize repulsive interactions between the ligands.
- Solvent effects: The solvent in which the coordination complex is dissolved can also affect its stability. Polar solvents, such as water, can interact strongly with the metal ion and ligands, stabilizing the complex. However, nonpolar solvents, such as benzene, may have little effect on the stability of the complex.
- Environmental factors: Factors such as temperature, pH, and the presence of other ions in solution can also affect the stability of coordination compounds. Changes in temperature or pH can lead to the dissociation of the complex, while the presence of other ions in solution can compete with the ligands for binding to the metal ion, destabilizing the complex.
Overall, the stability of coordination compounds is a complex interplay between a variety of factors, and understanding these factors is essential for designing and synthesizing effective and long-lasting coordination compounds.
Nomenclature of Coordination Compounds Stability
The nomenclature of coordination compounds is governed by a set of rules established by the International Union of Pure and Applied Chemistry (IUPAC). The nomenclature system is based on the names of the ligands and the central metal ion, with prefixes and suffixes used to indicate the number and nature of the ligands attached to the metal ion.
In terms of stability, the nomenclature system can provide some clues as to the stability of a coordination compound. For example, the suffix “-ate” is often used to indicate a negatively charged ligand, such as chloride or sulfate. In general, complexes with negatively charged ligands tend to be less stable than those with neutral or positively charged ligands, as the negatively charged ligands can repel each other, destabilizing the complex.
Another factor that can affect the stability of coordination compounds is the coordination number, which refers to the number of ligands attached to the central metal ion. Coordination compounds with coordination numbers that are close to the maximum possible coordination number for the metal ion, such as octahedral complexes with six ligands, tend to be more stable than those with lower coordination numbers.
The geometry of the coordination complex can also provide some clues as to its stability. For example, octahedral complexes, which have a high degree of symmetry, are generally more stable than complexes with lower symmetry, such as tetrahedral or square planar complexes. This is because the symmetrical geometry can minimize repulsive interactions between the ligands, making the complex more stable.
Overall, while the nomenclature system for coordination compounds does not directly provide information on stability, knowledge of the names and structures of the ligands and the metal ion can help to provide some insight into the likely stability of the complex. However, to fully understand the stability of a coordination compound, a range of other factors, such as the electronic and steric properties of the ligands and metal ion, must also be considered.
Case Study on Coordination Compounds Stability
One example of a case study on coordination compounds stability is the complex [Fe(CN)6]4-. This complex is a classic example of a highly stable coordination compound, due to several factors.
Firstly, the central metal ion, iron (Fe), has a partially filled d-orbital, which allows for strong interactions with the six cyanide ligands. The cyanide ligands are electron-rich and can form strong coordination bonds with the metal ion, stabilizing the complex.
Secondly, the geometry of the complex is highly symmetrical, with octahedral geometry, which minimizes repulsive interactions between the ligands. The ligands are arranged around the metal ion in a regular pattern, with bond angles of 90 and 180 degrees, maximizing the stability of the complex.
Thirdly, the negative charge of the complex is stabilized by the electronegative nature of the cyanide ligands, which can distribute the negative charge evenly around the complex, reducing electrostatic repulsion between the ligands.
Finally, the presence of the negative charge on the complex can also be stabilized by hydrogen bonding with water molecules, which can interact strongly with the partially negatively charged cyanide ligands.
Overall, the high stability of [Fe(CN)6]4- is due to a combination of factors, including the electron-rich nature of the ligands, the partially filled d-orbital of the metal ion, the highly symmetrical geometry, and the stabilizing effects of hydrogen bonding with water molecules. This complex has been extensively studied in the field of coordination chemistry and has provided important insights into the factors that influence the stability of coordination compounds.
White paper on Coordination Compounds Stability
Introduction:
Coordination compounds are a class of compounds in which a central metal ion is surrounded by ligands, which are typically molecules or ions that have at least one lone pair of electrons that can form a coordinate bond with the metal ion. The stability of coordination compounds is an important aspect of their chemistry, as it determines their ability to form and persist under different conditions. In this white paper, we will discuss the factors that influence the stability of coordination compounds, as well as their importance in various fields of chemistry.
Factors influencing stability:
The stability of coordination compounds depends on a number of factors, including the nature of the ligands, the electronic and steric properties of the metal ion, the geometry of the complex, and the presence of other species in the environment. One of the most important factors is the nature of the ligands, as this determines the strength of the coordination bond between the ligands and the metal ion. Ligands that are electron-rich, such as cyanide or ammonia, tend to form stronger bonds with the metal ion and therefore lead to more stable complexes.
The electronic and steric properties of the metal ion are also important factors. Metal ions that have a partially filled d-orbital, such as copper or iron, are generally more stable in coordination compounds than those with completely filled d-orbitals, as they can form stronger interactions with the ligands. Additionally, the size of the metal ion can influence the stability of the complex, with larger metal ions tending to form more stable complexes due to their ability to accommodate larger ligands.
The geometry of the complex is another important factor, with highly symmetrical complexes, such as octahedral complexes, being more stable than those with lower symmetry. This is because the symmetrical arrangement of ligands minimizes repulsive interactions between them, leading to a more stable complex.
The presence of other species in the environment can also influence the stability of coordination compounds. For example, the presence of other metal ions or anions can lead to the formation of competitive species that can displace the ligands from the metal ion, destabilizing the complex.
Importance in chemistry:
The stability of coordination compounds is important in a number of fields of chemistry, including biochemistry, catalysis, and materials science. In biochemistry, coordination compounds are involved in a variety of biological processes, such as the transport of oxygen in blood by hemoglobin, and the conversion of energy by cytochrome c oxidase. The stability of these compounds is essential for their biological function.
In catalysis, coordination compounds are used as catalysts in a range of chemical reactions, including the Haber process for the production of ammonia and the hydroformylation of alkenes. The stability of these complexes is important for their ability to catalyze reactions efficiently and selectively.
In materials science, coordination compounds are used as building blocks for the synthesis of a range of materials, such as metal-organic frameworks and coordination polymers. The stability of these compounds is important for their ability to form and persist under different conditions, which is essential for the development of functional materials.
Conclusion:
In conclusion, the stability of coordination compounds is an important aspect of their chemistry, as it determines their ability to form and persist under different conditions. The stability of these compounds depends on a number of factors, including the nature of the ligands, the electronic and steric properties of the metal ion, the geometry of the complex, and the presence of other species in the environment. The importance of coordination compounds in various fields of chemistry highlights the need for a thorough understanding of the factors that influence their stability.