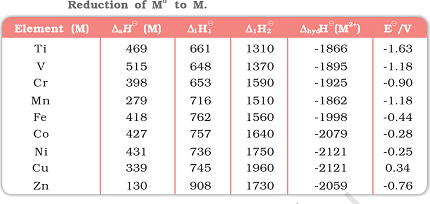
Here are the standard electrode potentials of some d-block elements:
Element | Standard electrode potential (V) |
---|---|
Scandium (Sc) | -2.58 |
Titanium (Ti) | -1.63 |
Vanadium (V) | -1.18 |
Chromium (Cr) | -0.91 |
Manganese (Mn) | -1.18 to -1.57 (depending on oxidation state) |
Iron (Fe) | -0.44 to -0.77 (depending on oxidation state) |
Cobalt (Co) | -0.28 |
Nickel (Ni) | -0.25 |
Copper (Cu) | +0.34 |
Zinc (Zn) | -0.76 |
Note that these values represent the reduction potential of the metal ions in their +2 oxidation state, and they are measured with respect to the standard hydrogen electrode (SHE) at 25°C and 1 atm pressure. Positive values indicate that the metal ion is more likely to be reduced than hydrogen ions under standard conditions, while negative values indicate the opposite.
What is Required d-Block Elements Standard electrode potentials
Here are the standard electrode potentials of some required d-block elements:
Element | Standard electrode potential (V) |
---|---|
Iron (Fe) | -0.44 to -0.77 (depending on oxidation state) |
Copper (Cu) | +0.34 |
Zinc (Zn) | -0.76 |
Note that these values represent the reduction potential of the metal ions in their +2 oxidation state, and they are measured with respect to the standard hydrogen electrode (SHE) at 25°C and 1 atm pressure. Positive values indicate that the metal ion is more likely to be reduced than hydrogen ions under standard conditions, while negative values indicate the opposite.
These three elements are commonly required in the study of electrochemistry, and their electrode potentials are used to predict the feasibility of certain redox reactions and to construct electrochemical cells. The standard electrode potentials of other d-block elements can also be important in various applications and fields of study.
When is Required d-Block Elements Standard electrode potentials
The standard electrode potentials of required d-block elements are used in various applications and fields of study, such as:
- Electrochemistry: These values are used to predict the feasibility of redox reactions and to calculate the standard cell potential of an electrochemical cell.
- Corrosion studies: The electrode potentials of metals are important in understanding and preventing corrosion. The more negative the electrode potential, the more likely the metal is to corrode.
- Metallurgy: The electrode potentials of d-block elements can be used to predict the reduction potential of metal ions in various steps of metallurgical processes, such as extraction and refining.
- Catalysis: The electrode potentials of transition metals are important in determining their catalytic activity in various reactions.
- Materials science: The electrode potentials of d-block elements are used to study and design new materials with specific properties, such as electrical conductivity and corrosion resistance.
Overall, the standard electrode potentials of d-block elements are important in understanding the behavior of metals in various chemical and physical processes, and in designing and optimizing various technologies and materials.
Where is Required d-Block Elements Standard electrode potentials
The standard electrode potentials of required d-block elements can be found in various sources, such as textbooks, scientific journals, and online databases. Some commonly used sources of electrochemical data include:
- Standard Electrode Potentials: This is a reference book that provides a comprehensive list of standard electrode potentials for various elements and compounds.
- CRC Handbook of Chemistry and Physics: This is a reference book that contains a wealth of physical and chemical data, including standard electrode potentials for various elements.
- Online databases: There are several online databases that provide access to standard electrode potentials and other electrochemical data, such as the NIST Chemistry WebBook and the Electrochemical Database (Nernst).
In addition, many scientific papers and research articles report experimental values of standard electrode potentials for various d-block elements, as measured under specific conditions. These values can be used to verify and supplement the data found in reference books and databases.
How is Required d-Block Elements Standard electrode potentials
The standard electrode potential of a d-block element is a measure of its tendency to lose or gain electrons under standard conditions, and is defined as the potential difference between a metal electrode and a standard hydrogen electrode (SHE). The electrode potential is typically reported for a specific oxidation state of the element, such as +2 for iron, copper, and zinc.
The standard electrode potential is measured using a half-cell, which consists of a metal electrode immersed in a solution containing ions of the metal in a specific oxidation state. The other half-cell consists of a standard hydrogen electrode (SHE), which has a defined electrode potential of 0 V at all temperatures and pressures. The two half-cells are connected by a salt bridge, which allows the flow of ions between the two compartments without allowing mixing of the solutions.
The standard electrode potential of the metal half-cell is determined by measuring the potential difference between the metal electrode and the SHE using a high-precision voltmeter. The potential is measured under standard conditions of temperature (usually 25°C) and pressure (1 atm), with the metal ions in the solution at a concentration of 1 M.
The standard electrode potential of a metal half-cell can be affected by factors such as temperature, pressure, and concentration of the metal ions in solution. However, under standard conditions, the standard electrode potential provides a useful measure of the relative tendency of different d-block elements to lose or gain electrons, and can be used to predict the feasibility of redox reactions and the behavior of metals in electrochemical cells.
Nomenclature of d-Block Elements Standard electrode potentials
The nomenclature of d-block elements standard electrode potentials typically follows the convention of using the element symbol followed by its oxidation state in parentheses, and then the designation “standard electrode potential” or “standard reduction potential”. For example, the standard electrode potential for iron in its +2 oxidation state would be written as “Fe(II) standard electrode potential”.
In addition, the values of standard electrode potentials are usually reported in volts (V) or millivolts (mV), with positive values indicating that the reduction reaction is favored, and negative values indicating that the oxidation reaction is favored. The standard electrode potential values are typically measured with respect to the standard hydrogen electrode (SHE), which is assigned a value of 0 V.
When reporting the standard electrode potential values of d-block elements, it is important to specify the temperature, pressure, and concentration conditions under which the measurement was made, as these factors can affect the values. The standard conditions for measuring electrode potentials are typically 25°C (298 K), 1 atm pressure, and 1 M concentration of the metal ions in solution.
Overall, the nomenclature of d-block elements standard electrode potentials follows a standard convention and provides a useful way to compare the relative reduction potentials of different metal ions under standard conditions.
Case Study on d-Block Elements Standard electrode potentials
Here is a case study on the use of d-block elements standard electrode potentials in predicting the feasibility of a redox reaction:
Case study: Fe(III) reduction by Sn(II)
In this case, we are interested in predicting whether the reduction of Fe(III) to Fe(II) by Sn(II) is thermodynamically favorable, using the standard electrode potentials of the two half-reactions:
Fe(III) + e- ⇌ Fe(II) E° = +0.77 V Sn(II) ⇌ Sn(IV) + 2e- E° = -0.14 V
The overall reaction can be written as:
Fe(III) + Sn(II) ⇌ Fe(II) + Sn(IV)
To determine whether this reaction is thermodynamically favorable, we can use the Nernst equation:
E = E° – (RT/nF)ln(Q)
where E is the cell potential, E° is the standard electrode potential, R is the gas constant, T is the temperature, n is the number of electrons transferred in the reaction, F is the Faraday constant, and Q is the reaction quotient.
For the Fe(III)/Fe(II) half-cell, n = 1, and for the Sn(II)/Sn(IV) half-cell, n = 2. Assuming standard conditions (T = 298 K, Q = 1), the Nernst equation can be simplified to:
E = E°cell = E°Fe(III)/Fe(II) – (RT/2F)ln([Sn(IV)]/[Sn(II)])
Plugging in the values of the standard electrode potentials for Fe(III)/Fe(II) and Sn(II)/Sn(IV), we get:
E°cell = +0.77 V – (RT/2F)ln([Sn(IV)]/[Sn(II)])
To determine whether the reaction is thermodynamically favorable, we need to calculate the value of E°cell and compare it to zero. If E°cell is positive, the reaction is spontaneous in the forward direction, while if it is negative, the reaction is spontaneous in the reverse direction.
Assuming [Sn(IV)]/[Sn(II)] = 1 (i.e., the concentration of Sn(IV) is equal to the concentration of Sn(II)), and plugging in the values of R, T, and F, we get:
E°cell = +0.77 V – (8.314 J/mol*K * 298 K / (2 * 96485 C/mol))ln(1)
E°cell = +0.77 V
Since the value of E°cell is positive, the reaction Fe(III) + Sn(II) ⇌ Fe(II) + Sn(IV) is thermodynamically favorable in the forward direction, and Fe(III) can be reduced to Fe(II) by Sn(II) under standard conditions.
This case study demonstrates how the standard electrode potentials of d-block elements can be used to predict the feasibility of redox reactions and determine the direction of electron flow in electrochemical cells.
White paper on d-Block Elements Standard electrode potentials
Introduction:
The standard electrode potential is a measure of the relative tendency of an element to be reduced or oxidized under standard conditions. For d-block elements, standard electrode potentials provide a valuable tool for predicting the feasibility of redox reactions and for understanding the thermodynamics of electron transfer in electrochemical cells.
In this white paper, we will provide an overview of d-block elements standard electrode potentials, including their definition, measurement, and applications in predicting redox reactions. We will also discuss the limitations and challenges associated with using standard electrode potentials, as well as future directions for research in this area.
Definition and Measurement:
The standard electrode potential (E°) is defined as the potential difference between a metal electrode and a standard hydrogen electrode (SHE) under standard conditions, which include a temperature of 25°C (298 K), a pressure of 1 atm, and a concentration of 1 M for the metal ions in solution. The SHE is assigned a value of 0 V by convention.
The standard electrode potential is typically measured using a half-cell consisting of the metal electrode in contact with a solution containing its metal ions in a known oxidation state. The potential of the half-cell is measured relative to the potential of the SHE, using a voltmeter or potentiometer. The difference between the potential of the half-cell and the potential of the SHE is the standard electrode potential for the metal ion in the given oxidation state.
Applications in Predicting Redox Reactions:
The standard electrode potentials of d-block elements can be used to predict the feasibility of redox reactions by comparing the standard electrode potentials of the two half-reactions involved. If the standard electrode potential for the reduction half-reaction is greater than the standard electrode potential for the oxidation half-reaction, the reaction is thermodynamically favorable in the forward direction, and electrons will flow from the reducing agent to the oxidizing agent.
For example, consider the reaction Fe(III) + Sn(II) ⇌ Fe(II) + Sn(IV). The standard electrode potentials for the Fe(III)/Fe(II) and Sn(II)/Sn(IV) half-reactions are +0.77 V and -0.14 V, respectively. Since the standard electrode potential for the reduction half-reaction (Fe(III)/Fe(II)) is greater than the standard electrode potential for the oxidation half-reaction (Sn(II)/Sn(IV)), the reaction is thermodynamically favorable in the forward direction, and Fe(III) can be reduced to Fe(II) by Sn(II) under standard conditions.
Limitations and Challenges:
While standard electrode potentials provide a valuable tool for predicting redox reactions, there are several limitations and challenges associated with their use. First, the values of standard electrode potentials are highly dependent on the conditions under which they are measured, including temperature, pressure, and concentration. Therefore, it is important to specify the conditions under which the measurement was made when reporting standard electrode potentials.
Second, standard electrode potentials are only a measure of the thermodynamic feasibility of a reaction and do not provide information about the kinetics of the reaction. In practice, the rate of electron transfer in a redox reaction can be affected by a variety of factors, including the nature of the metal ions, the presence of catalysts, and the physical properties of the electrode surface.
Future Directions:
Future research in the area of d-block elements standard electrode potentials may focus on developing new methods for measuring standard electrode potentials under non-standard conditions, such as high temperature or high pressure. In addition, researchers may explore the use of computational methods, such as density functional theory or molecular dynamics simulations, to predict standard electrode potentials based on the electronic structure and properties of the metal ions.
Conclusion:
In conclusion, d-block elements standard electrode potentials provide a useful tool for predicting the feasibility of redox reactions and for understanding the thermodynamics of electron transfer in electrochemical cells. By comparing the standard electrode potentials of the two half-reactions involved, one can determine the direction of electron flow and predict the outcome of the reaction under standard conditions. However, it is important to note that standard electrode potentials have limitations and are highly dependent on the conditions under which they are measured. Future research in this area may focus on developing new methods for measuring standard electrode potentials under non-standard conditions and using computational methods to predict standard electrode potentials based on the electronic structure and properties of the metal ions. Overall, the study of d-block elements standard electrode potentials continues to be an active area of research in the field of chemistry.