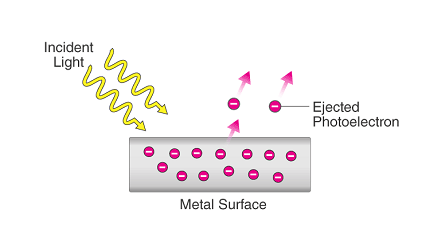
The photoelectric effect is the phenomenon of the emission of electrons from a material when it is exposed to electromagnetic radiation, such as visible light or ultraviolet light. This effect was first observed by Heinrich Hertz in 1887 and was explained by Albert Einstein in 1905, for which he received the Nobel Prize in Physics in 1921.
The photoelectric effect occurs when a photon of light collides with an electron in a material, imparting enough energy to the electron to overcome the binding energy that holds it in place. The electron is then emitted from the material, creating a flow of electric current. The energy of the photon must be greater than the binding energy of the electron in order for the photoelectric effect to occur.
The photoelectric effect has many applications, including in solar cells, photodiodes, and photoelectric sensors. It also played a key role in the development of quantum mechanics, which is the branch of physics that deals with the behavior of matter and energy at the atomic and subatomic level.
What is Required Photoelectric Effect
The photoelectric effect occurs when a photon of light collides with an electron in a material and imparts enough energy to the electron to overcome the binding energy that holds it in place, causing the electron to be ejected from the material. In order for the photoelectric effect to occur, several conditions must be met:
- Energy of the Photon: The energy of the photon of light must be greater than the binding energy of the electron in the material. If the energy of the photon is not sufficient, the electron will not be able to overcome the binding energy and the photoelectric effect will not occur.
- Nature of the Material: The material must be a metal or a semiconductor with a suitable bandgap. Materials that have high work functions (i.e. require more energy to remove an electron from the material) are more likely to exhibit the photoelectric effect.
- Intensity of Light: The intensity of the light, or the number of photons per unit area per unit time, affects the number of electrons that are emitted. However, the energy of the photons is what determines the maximum kinetic energy of the emitted electrons.
- Angle of Incidence: The angle at which the light strikes the material can also affect the number of emitted electrons. At certain angles, the number of electrons emitted can be increased due to the interaction between the electrons and the surface of the material.
These factors collectively contribute to the probability and strength of the photoelectric effect.
When is Required Photoelectric Effect
The photoelectric effect is a fundamental process that occurs whenever light interacts with matter, and it has many practical applications in modern technology. Here are some examples of when the photoelectric effect is required:
- Solar Energy: The photoelectric effect is essential for solar cells, which convert sunlight into electricity. The cells are made of semiconductor materials that have a suitable bandgap to allow for the absorption of photons and the generation of electron-hole pairs. When the electrons are collected, they create an electric current.
- Photodetectors: The photoelectric effect is also used in photodetectors, which are devices that detect and measure light. In photodiodes, for example, the incoming photons create electron-hole pairs, which can be detected as a current flow.
- X-ray Imaging: X-ray imaging relies on the photoelectric effect to produce images of the body. X-rays are high-energy photons that are absorbed by the body’s tissues, causing the emission of electrons that can be detected and used to create an image.
- Atomic Spectroscopy: The photoelectric effect is used in atomic spectroscopy to analyze the elemental composition of a sample. When the sample is irradiated with light of a specific wavelength, the electrons in the sample absorb the photons and are ejected, creating a measurable signal that can be used to identify the elements present.
Overall, the photoelectric effect is an important process that has many practical applications in various fields, including energy, medicine, and materials science.
Where is Required Photoelectric Effect
The photoelectric effect is a fundamental process that occurs whenever light interacts with matter, and it is a universal phenomenon that can occur in any material that absorbs photons of light. Here are some examples of where the photoelectric effect is required:
- Solar Energy: Solar panels use the photoelectric effect to convert sunlight into electricity. The panels are made of semiconductor materials such as silicon, which can absorb photons and generate electron-hole pairs. The electrons are then collected and used to create an electric current.
- Photography: The photoelectric effect is used in photography to create images. When light strikes a photographic film, the photons cause the emission of electrons, which create a latent image that can be developed to produce a visible image.
- X-ray Imaging: X-ray imaging relies on the photoelectric effect to produce images of the body. X-rays are high-energy photons that are absorbed by the body’s tissues, causing the emission of electrons that can be detected and used to create an image.
- Atomic Spectroscopy: The photoelectric effect is used in atomic spectroscopy to analyze the elemental composition of a sample. When the sample is irradiated with light of a specific wavelength, the electrons in the sample absorb the photons and are ejected, creating a measurable signal that can be used to identify the elements present.
Overall, the photoelectric effect is a universal phenomenon that can occur in any material that absorbs photons of light, and it has many practical applications in various fields, including energy, medicine, and materials science.
How is Required Photoelectric Effect
The photoelectric effect occurs when a photon of light interacts with matter, such as a metal or a semiconductor, and transfers its energy to an electron within the material. Here’s a step-by-step description of how the photoelectric effect occurs:
- A photon of light strikes the surface of a material, such as a metal or a semiconductor.
- The photon transfers its energy to an electron within the material, giving the electron enough energy to overcome the binding energy that holds it in place.
- The electron is ejected from the material, creating a flow of electric current.
- The kinetic energy of the ejected electron is given by the difference between the energy of the incident photon and the binding energy of the electron in the material.
- If the material is a metal, the ejected electron is called a photoelectron. If the material is a semiconductor, the ejected electron-hole pair is called an exciton.
- The probability of the photoelectric effect depends on several factors, including the energy of the incident photon, the nature of the material, the angle of incidence, and the intensity of the light.
The photoelectric effect is a fundamental process that has many practical applications, such as in solar cells, photodetectors, and X-ray imaging. Understanding how the photoelectric effect works is important for developing new technologies and advancing our understanding of the behavior of matter and energy at the atomic and subatomic level.
Structures of Photoelectric Effect
The photoelectric effect involves the interaction between light and matter, resulting in the ejection of electrons from a material. The process occurs in various structures, including metals and semiconductors. Here are some details about the structures of the photoelectric effect:
- Metals: In metals, the valence electrons are loosely bound and can easily move within the material. When a photon of light with energy greater than the work function of the metal strikes the surface, it transfers its energy to an electron, which can be ejected from the metal. This ejected electron is called a photoelectron.
- Semiconductors: In semiconductors, the valence electrons are bound more tightly than in metals, and there is a bandgap between the valence band and the conduction band. When a photon of light with energy greater than the bandgap strikes the surface, it generates an electron-hole pair, consisting of a negatively charged electron and a positively charged hole. The electron and the hole can move freely within the semiconductor and contribute to the flow of electric current.
- Photocathodes: Photocathodes are specialized materials used in photomultiplier tubes and other detectors to convert photons into electrons. They are typically made of metals with a low work function, such as cesium, which allows for efficient ejection of photoelectrons.
- Photovoltaic Cells: Photovoltaic cells, also known as solar cells, are made of semiconducting materials such as silicon or cadmium telluride. When a photon of light strikes the surface of the cell, it creates an electron-hole pair, which can be separated by an electric field within the cell and collected to create an electric current.
In summary, the photoelectric effect occurs in various structures, including metals, semiconductors, photocathodes, and photovoltaic cells. The specific structure and material properties determine the efficiency and mechanism of the process.
Case Study on Photoelectric Effect
One example of the photoelectric effect in action is the development of photovoltaic cells, which are devices that convert sunlight directly into electrical energy. Here is a case study on the photoelectric effect in the development of photovoltaic cells:
Background: Photovoltaic cells, also known as solar cells, are made of semiconductor materials, such as silicon, that absorb photons of light and generate electric current. The photoelectric effect is the fundamental process that allows photovoltaic cells to work. When photons of sunlight strike the surface of the cell, they transfer their energy to electrons within the semiconductor material, causing them to become excited and move from the valence band to the conduction band. The excited electrons are then collected by an external circuit to create an electric current.
Case Study: The development of photovoltaic cells began in the 19th century with the discovery of the photoelectric effect by Heinrich Hertz in 1887. The first photovoltaic cell was made of selenium, a material that exhibited photoconductivity. In 1954, Bell Labs developed the first practical silicon-based solar cell, which had an efficiency of 4.5%.
Since then, the efficiency of photovoltaic cells has increased significantly, thanks to ongoing research and development. One approach to improving efficiency is to optimize the bandgap of the semiconductor material to match the solar spectrum. Another approach is to use multi-junction cells, which consist of multiple layers of semiconductors with different bandgaps to capture a wider range of solar wavelengths.
Recent advancements in materials science and nanotechnology have also led to the development of new types of photovoltaic cells, such as perovskite solar cells and quantum dot solar cells. These materials exhibit unique properties that allow for improved efficiency and lower production costs.
Conclusion: The photoelectric effect is a fundamental process that allows photovoltaic cells to convert sunlight into electrical energy. Ongoing research and development have led to significant improvements in the efficiency and cost-effectiveness of photovoltaic cells. As the world continues to shift towards renewable energy sources, the photoelectric effect and photovoltaic cells will play an increasingly important role in meeting the growing demand for clean energy.
White paper on Photoelectric Effect
Introduction:
The photoelectric effect is a fundamental phenomenon in physics, which refers to the ejection of electrons from a material when it is exposed to electromagnetic radiation, such as light. It was first observed by Heinrich Hertz in 1887 and later explained by Albert Einstein in 1905, for which he received the Nobel Prize in Physics in 1921. The photoelectric effect is the basis for many technologies, including photovoltaic cells, photomultiplier tubes, and image sensors. In this white paper, we will discuss the photoelectric effect, its principles, applications, and future prospects.
Principles of the Photoelectric Effect:
The photoelectric effect is the process of the ejection of electrons from a material when it is exposed to electromagnetic radiation. The energy of the radiation must be greater than or equal to the work function of the material for the electrons to be ejected. The work function is the energy required to remove an electron from the surface of the material. If the energy of the radiation is less than the work function, the electrons will not be ejected.
The photoelectric effect can be explained by the quantum theory of light, which describes light as consisting of photons, each with a specific energy and wavelength. When a photon strikes the surface of a material, it transfers its energy to an electron in the material. If the energy of the photon is greater than the work function of the material, the electron will be ejected from the surface. The ejected electron is called a photoelectron, and its energy is equal to the energy of the photon minus the work function of the material.
Applications of the Photoelectric Effect:
The photoelectric effect has many practical applications, including photovoltaic cells, photomultiplier tubes, and image sensors.
Photovoltaic cells, also known as solar cells, convert sunlight directly into electrical energy. They consist of semiconductor materials, such as silicon, which absorb photons of light and generate electric current. The photoelectric effect is the fundamental process that allows photovoltaic cells to work. When photons of sunlight strike the surface of the cell, they transfer their energy to electrons within the semiconductor material, causing them to become excited and move from the valence band to the conduction band. The excited electrons are then collected by an external circuit to create an electric current.
Photomultiplier tubes are devices used to detect and amplify the intensity of light. They consist of a photocathode, which is a material that emits electrons when struck by photons of light, and a series of dynodes, which are metal electrodes that amplify the number of electrons. The photoelectric effect is the process that allows the photocathode to emit electrons when struck by photons of light.
Image sensors are electronic devices used in digital cameras and other imaging applications to convert optical images into electrical signals. They consist of an array of photodiodes, which are semiconductor devices that convert photons of light into electrical current. The photoelectric effect is the process that allows the photodiodes to convert photons of light into electrical current.
Future Prospects:
The photoelectric effect has already revolutionized the way we generate and use energy, and it will continue to play an increasingly important role in the future. The development of new materials, such as perovskites and quantum dots, is expected to lead to significant improvements in the efficiency and cost-effectiveness of photovoltaic cells. The use of photovoltaic cells and other photoelectric devices is also expected to increase as the world shifts towards renewable energy sources to address the challenges of climate change and energy security.
Conclusion:
The photoelectric effect is a fascinating phenomenon that has led to the development of many important technologies, including photovoltaic cells, photomultiplier tubes, and image sensors. The principles of the photoelectric effect are based on the quantum theory of light, which describes light as consisting of photons with specific energies and wavelengths. When photons strike the surface of a material, they transfer their energy to electrons within the material, causing them to become excited and potentially leading to the ejection of electrons from the surface. The photoelectric effect has already revolutionized the way we generate and use energy, and it is expected to continue to play a vital role in the future, especially as the world shifts towards renewable energy sources.