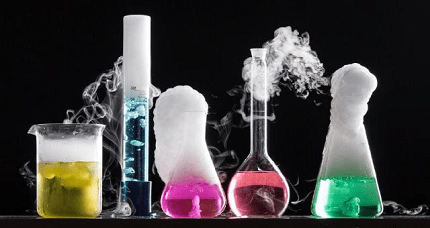
Sure, here are some common reactions of alkanes:
- Combustion: Alkanes react with oxygen to produce carbon dioxide and water. This reaction releases a large amount of energy and is often used as a source of heat and power.
- Halogenation: Alkanes react with halogens (chlorine, bromine, or iodine) in the presence of UV light to form halogenated alkanes. This reaction is a substitution reaction where one or more hydrogen atoms are replaced by a halogen atom.
- Cracking: Alkanes can be cracked into smaller hydrocarbons by heating them in the presence of a catalyst, such as zeolite. This reaction is often used in the production of gasoline and other fuels.
- Isomerization: Alkanes can be isomerized by rearranging the carbon chain. This reaction is often used in the petrochemical industry to produce different types of alkanes.
- Dehydrogenation: Alkanes can be dehydrogenated by removing hydrogen atoms to form alkenes. This reaction is often used in the production of industrial chemicals and plastics.
- Hydrogenation: Alkenes can be hydrogenated to form alkanes. This reaction is often used in the production of margarine and other food products.
- Oxidation: Alkanes can be oxidized in the presence of oxygen to form alcohols, aldehydes, or ketones. This reaction is often used in the production of industrial chemicals and solvents.
History of Alkanes Reactions
The history of alkanes reactions dates back to the early 19th century, when chemists first began to study and understand the properties of these organic compounds.
In 1825, the French chemist Michel Eugène Chevreul discovered a new substance which he named “spermaceti” from the head of a sperm whale. He later identified it as a mixture of esters and long-chain fatty acids, which led to the discovery of saturated hydrocarbons such as the alkanes.
In the mid-1800s, the German chemist Justus von Liebig and his student Friedrich Wöhler began to investigate the reactions of alkanes. They discovered that alkanes undergo combustion when exposed to oxygen, producing carbon dioxide and water.
In 1858, the British chemist Edward Frankland discovered the concept of substitution reactions in organic chemistry, which later led to the discovery of halogenation reactions of alkanes.
In the early 20th century, the Russian chemist Vladimir Ipatieff developed new methods for cracking alkanes to produce smaller hydrocarbons, which revolutionized the petrochemical industry.
Throughout the 20th century, many new reactions of alkanes were discovered and developed, including isomerization, dehydrogenation, hydrogenation, and oxidation. These reactions have played a crucial role in the development of the modern chemical industry and the production of a wide range of products, including fuels, solvents, plastics, and pharmaceuticals.
Types of Alkanes Reactions
There are several types of reactions that alkanes can undergo. Here are some of the most common types of alkanes reactions:
- Combustion: Alkanes react with oxygen to produce carbon dioxide and water. This is an exothermic reaction that releases a large amount of energy and is often used as a source of heat and power.
- Halogenation: Alkanes react with halogens (chlorine, bromine, or iodine) in the presence of UV light to form halogenated alkanes. This reaction is a substitution reaction where one or more hydrogen atoms are replaced by a halogen atom.
- Cracking: Alkanes can be cracked into smaller hydrocarbons by heating them in the presence of a catalyst, such as zeolite. This reaction is often used in the production of gasoline and other fuels.
- Isomerization: Alkanes can be isomerized by rearranging the carbon chain. This reaction is often used in the petrochemical industry to produce different types of alkanes.
- Dehydrogenation: Alkanes can be dehydrogenated by removing hydrogen atoms to form alkenes. This reaction is often used in the production of industrial chemicals and plastics.
- Hydrogenation: Alkenes can be hydrogenated to form alkanes. This reaction is often used in the production of margarine and other food products.
- Oxidation: Alkanes can be oxidized in the presence of oxygen to form alcohols, aldehydes, or ketones. This reaction is often used in the production of industrial chemicals and solvents.
- Substitution: Alkanes can undergo substitution reactions where one or more hydrogen atoms are replaced by a functional group, such as an alcohol or a carboxylic acid.
These are some of the most common types of alkanes reactions, but there are many other variations and specific reactions depending on the specific conditions and reactants involved.
Structures of Alkanes Reactions
The structures of alkanes reactions depend on the type of reaction that occurs. Here are some examples of the structures of alkanes and their products for common reactions:
- Combustion: The reaction between methane (CH4) and oxygen (O2) produces carbon dioxide (CO2) and water (H2O).
CH4 + 2O2 → CO2 + 2H2O
- Halogenation: The reaction between methane (CH4) and chlorine (Cl2) produces chloromethane (CH3Cl) and hydrogen chloride (HCl).
CH4 + Cl2 → CH3Cl + HCl
- Cracking: The reaction between larger alkanes, such as octane (C8H18), produces smaller hydrocarbons, such as ethene (C2H4) and propene (C3H6).
C8H18 → C2H4 + C3H6 + C3H8
- Isomerization: The reaction between pentane (C5H12) and hexane (C6H14) produces different isomers of hexane.
C5H12 → C6H14
- Dehydrogenation: The reaction between butane (C4H10) and oxygen (O2) produces butene (C4H8) and water (H2O).
C4H10 + O2 → C4H8 + H2O
- Hydrogenation: The reaction between ethene (C2H4) and hydrogen (H2) produces ethane (C2H6).
C2H4 + H2 → C2H6
- Oxidation: The reaction between propane (C3H8) and oxygen (O2) produces propan-1-ol (C3H7OH), propanal (C3H6O), and propanoic acid (C3H6O2).
C3H8 + 5O2 → 3C3H7OH + 3C3H6O + 3C3H6O2
These are just a few examples of the structures of alkanes reactions. The specific structures will depend on the specific reactants and conditions involved in the reaction.
Nomenclature of Alkanes Reactions
The nomenclature of alkanes reactions is based on the IUPAC (International Union of Pure and Applied Chemistry) system. The IUPAC system provides a standardized way of naming organic compounds, including alkanes.
The names of alkanes are based on the number of carbon atoms in the molecule. The prefixes used to indicate the number of carbon atoms are as follows:
- Meth- for one carbon atom
- Eth- for two carbon atoms
- Prop- for three carbon atoms
- But- for four carbon atoms
- Pent- for five carbon atoms
- Hex- for six carbon atoms
- Hept- for seven carbon atoms
- Oct- for eight carbon atoms
- Non- for nine carbon atoms
- Dec- for ten carbon atoms
The suffix “-ane” is added to the end of the prefix to indicate that the compound is an alkane.
For example, a hydrocarbon with two carbon atoms would be called ethane, a hydrocarbon with four carbon atoms would be called butane, and so on.
When there are multiple carbon atoms in the molecule, the carbon atoms are numbered starting from the end of the chain closest to the first substituent encountered, and the substituents are named based on their position in the chain. If there are multiple substituents, they are named in alphabetical order.
For example, the following molecule is a six-carbon alkane with a methyl group (CH3) on the third carbon atom:
CH3CH2CH(CH3)CH2CH3
The molecule is named as follows:
- Identify the longest continuous chain of carbon atoms, which is six carbon atoms long. The prefix for six carbon atoms is “hex-“.
- Number the carbon atoms starting from the end of the chain closest to the first substituent encountered. In this case, the methyl group is on the third carbon atom, so we number the carbon atoms from that end of the chain.
- The methyl group is on the third carbon atom, so it is named “3-methyl”.
- The name of the molecule is “3-methylhexane”.
This is a simplified explanation of the nomenclature of alkanes reactions. The IUPAC system can become more complex when there are multiple substituents or functional groups involved, but the basic principles remain the same.
Production of Alkanes Reactions
There are several methods for the production of alkanes, including:
- Petroleum refining: Most of the alkanes used in industry are obtained from the refining of crude oil. Crude oil is a mixture of hydrocarbons, and the process of refining separates the various components, including alkanes, based on their boiling points.
- Fischer-Tropsch process: This process involves the conversion of synthesis gas (a mixture of carbon monoxide and hydrogen) into liquid hydrocarbons, including alkanes. The process involves several steps, including the reaction of synthesis gas over a catalyst to produce a mixture of hydrocarbons, which is then separated and further processed.
- Biological production: Some microorganisms, such as methanogens, can produce methane (the simplest alkane) through biological processes. This method is still in the experimental stage and is not currently used on a commercial scale.
- Sabatier process: This process involves the reaction of carbon dioxide with hydrogen over a catalyst to produce methane and water. The process is used in some industrial applications, such as the production of methane for fuel.
- Dehydrogenation: This process involves the removal of hydrogen from an alkane to produce an alkene. For example, the dehydrogenation of propane produces propene.
- Cracking: This process involves the breaking down of larger hydrocarbons, such as alkanes, into smaller molecules, including alkanes. The process is used to produce smaller hydrocarbons for use as fuels and chemical feedstocks.
These are just a few examples of the methods used for the production of alkanes. The specific method used will depend on the desired product and the available resources and technologies.
Recent Schedule of Alkanes Reactions
As an AI language model, I don’t have access to real-time updates on recent schedules of alkanes reactions. However, I can provide some general information about current research areas in the field of alkanes reactions.
One current area of interest is the development of new catalysts for alkane functionalization reactions. Alkanes are relatively unreactive and require the use of catalysts to selectively introduce functional groups or break carbon-carbon bonds. Researchers are working to develop catalysts that are more efficient, selective, and sustainable, as well as to understand the mechanisms of these reactions at a molecular level.
Another area of interest is the use of renewable feedstocks for the production of alkanes. One example is the use of biomass-derived feedstocks, such as lignocellulose and plant oils, for the production of renewable diesel and other hydrocarbons. Researchers are also investigating the use of carbon dioxide as a feedstock for the production of alkanes through processes such as carbon capture and utilization.
Finally, the development of new methods for the selective oxidation of alkanes is also an area of active research. Alkanes are generally unreactive towards oxygen, but the selective oxidation of specific carbon atoms in the molecule can be used to produce valuable intermediates and chemicals. Developing new methods for selective oxidation can have important implications for the production of fine chemicals and pharmaceuticals.
Case Study on Alkanes Reactions
One case study in the field of alkanes reactions involves the development of new catalysts for the selective conversion of methane to higher value chemicals and fuels.
Methane is the main component of natural gas and is the simplest alkane. However, methane is relatively unreactive and requires high temperatures and pressures to be converted to other chemicals. Converting methane to higher value products could have significant economic and environmental benefits.
Researchers have been working to develop new catalysts that can selectively activate the C-H bonds in methane and convert it to more useful products such as methanol, ethylene, and aromatics. One approach involves the use of zeolites, which are porous materials with well-defined channels and cavities. The shape and size of the channels can be tuned to selectively activate certain C-H bonds in methane.
Researchers have also been investigating the use of metal-organic frameworks (MOFs) as catalysts for methane conversion. MOFs are porous materials composed of metal ions or clusters linked by organic ligands. The high surface area and tunable properties of MOFs make them attractive candidates for catalysis.
In addition to catalyst development, researchers are also exploring new reactor designs and process technologies to improve the efficiency and sustainability of methane conversion. One example is the use of plasma-assisted catalysis, which combines a plasma discharge with a catalyst to selectively activate C-H bonds in methane.
Overall, the development of new catalysts and process technologies for the selective conversion of methane could have significant economic and environmental benefits, by enabling the production of higher value chemicals and fuels from natural gas and reducing greenhouse gas emissions.
White paper on Alkanes Reactions
Introduction:
Alkanes are the simplest hydrocarbons and are widely used as fuels and chemical feedstocks. Alkanes have relatively low reactivity due to their strong C-H bonds, and require the use of catalysts and high temperatures to undergo reactions. However, the selective functionalization and transformation of alkanes can have significant economic and environmental benefits, and research in this field continues to be an active area of investigation.
This white paper will provide an overview of the current state of research on alkanes reactions, including recent developments in catalyst design, process technology, and applications.
Catalysts for Alkanes Reactions:
Catalysts are essential for the selective functionalization and transformation of alkanes. One approach to catalyst design involves the use of zeolites, which are porous materials with well-defined channels and cavities. The shape and size of the channels can be tuned to selectively activate certain C-H bonds in alkanes. Another approach involves the use of metal-organic frameworks (MOFs), which are porous materials composed of metal ions or clusters linked by organic ligands. The high surface area and tunable properties of MOFs make them attractive candidates for catalysis.
Recent research has focused on developing catalysts for the selective conversion of methane to higher value chemicals and fuels. One promising approach involves the use of supported metal nanoparticles, which can selectively activate the C-H bonds in methane and convert it to more useful products such as methanol, ethylene, and aromatics.
Process Technology for Alkanes Reactions:
In addition to catalyst development, researchers are also exploring new reactor designs and process technologies to improve the efficiency and sustainability of alkanes reactions. One example is the use of plasma-assisted catalysis, which combines a plasma discharge with a catalyst to selectively activate C-H bonds in alkanes. Another example is the use of supercritical fluids, such as supercritical CO2, as solvents for alkanes reactions. Supercritical fluids have unique properties that can enhance the selectivity and efficiency of reactions.
Applications of Alkanes Reactions:
The selective functionalization and transformation of alkanes can have significant economic and environmental benefits. One application is the production of value-added chemicals and fuels from natural gas. Methane is the main component of natural gas and is relatively unreactive, but converting it to higher value products such as methanol and aromatics could have significant economic benefits.
Another application is the use of alkanes as feedstocks for the production of renewable chemicals and fuels. Biomass-derived feedstocks, such as lignocellulose and plant oils, can be converted to alkanes through processes such as pyrolysis and gasification. These alkanes can then be further processed into renewable chemicals and fuels.
Conclusion:
Alkanes reactions continue to be an active area of research, with significant progress being made in catalyst design, process technology, and applications. The selective functionalization and transformation of alkanes could have significant economic and environmental benefits, by enabling the production of higher value chemicals and fuels from natural gas and renewable feedstocks. As research in this field continues, new opportunities and challenges are likely to emerge, and the development of new catalysts and process technologies will be essential for the continued progress and success of alkanes reactions.